Article
Debris Flow Hazard Assessment in District Chitral, Eastern Hindu Kush, Pakistan
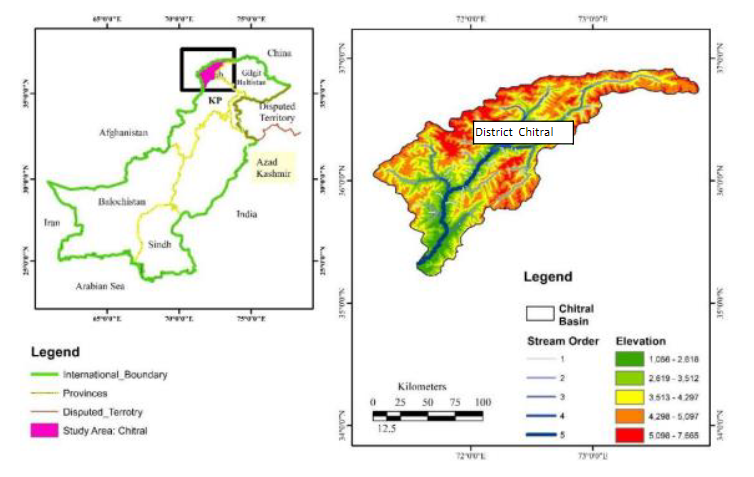
Downloads
Download

This work is licensed under a Creative Commons Attribution 4.0 International License.
Copyright
The authors shall retain the copyright of their work but allow the Publisher to publish, copy, distribute, and convey the work.
License
Prevention and Treatment of Natural Disasters (PTND) publishes accepted manuscripts under Creative Commons Attribution 4.0 International (CC BY 4.0). Authors who submit their papers for publication by Prevention and Treatment of Natural Disasters (PTND) agree to have the CC BY 4.0 license applied to their work, and that anyone is allowed to reuse the article or part of it free of charge for any purpose, including commercial use. As long as the author and original source is properly cited, anyone may copy, redistribute, reuse and transform the content.
The aim of this study is debris flow risk zonation using geological and hydrometeorological indicators in district Chitral, Hindu Kush Region Northwest Pakistan. The research is based on secondary data. Multi criteria Analysis (MCA) in Geographical Information System (GIS) environment was used to achieve the objective of the study. The geological and hydrometeorological parameters were analyzed by making five classes of each parameter. The classes are ranked as most favorable and least favorable with numerical weights. The weights were assigned in accordance to their importance in debris flow occurrence. Then weighted overlay analysis techniques were applied to develop composite map representing the importance of each factor. Debris flow risk zonation map was resulted into four classes very high risk zones, high risk zone, moderate zone, low risk zone. The geology of the study area is diverse with frequents earthquakes. Similarly the forest cover is decreasing due to anthropogenic activities. The area is also characterized by long cold winters with frost action. These factors are destabilizing the slope. During summer season rain storm event results high surface runoff and peak discharge in the perennial and non-perennial channels which results flood and debris flow. These events result human life loss and disruption. The main villages located in very high risk zone are Mulkoh, Mastuj, Reshun, Shegram, Terich Gol, Rogar, Asurat, Boni, Brep and Rech Tockhow. They have been frequently affected by hazard in the past decade. Out of the total area, very high risk zone is expanded over 8%, high risk zone is expanded over16%, moderate risk zone is 29% and the rest is low risk zone. This study has highlighted the risk zones which will help disaster management authorities and policy makers to reduce the risk of debris flow in future.
Keywords:
Debris flow Hazard MCA GIS Slope Lithology Chitral.References
- Mahmood S, Khan AH, Mayo SM (2016a) Exploring underlying causes and assessing damages of 2010 flash flood in the upper zone of Panjkora River. Nat Hazards 83(2):1213–1227.
- Mahmood S, Khan AH, Ullah S (2016b) Assessment of 2010 flash flood causes and associated damages in Dir Valley, Khyber Pakhtunkhwa Pakistan. Int J Disaster Risk Reduct 16:215–223
- Gautam, P., Kubota, T., Sapkota, L. M., and Shinohara, Y. (2021). Landslide susceptibility mapping with GIS in high mountain area of Nepal: a comparison of four methods. Environ. Earth Sci. 80, 359
- Rahman, G., Rahman, A.-U., Ullah, S., Miandad, M., and Collins, A. E. (2019). Spatial analysis of landslide susceptibility using failure rate approach in the Hindu Kush region, Pakistan. J. Earth Syst. Sci. 128, 59.
- Archetti, R., & Lamberti, A. (2003). Assessment of risk due to debris flow events. Natural Hazards Review, 4(3), 115-125.
- Meten, M., PrakashBhandary, N., & Yatabe, R. (2015). Effect of landslide factor combinations on the prediction accuracy of landslide susceptibility maps in the Blue Nile Gorge of Central Ethiopia. Geoenvironmental Disasters, 2(1), 1-17.
- Dowling, C. A., & Santi, P. M. (2014). Debris flows and their toll on human life: a global analysis of debris-flow fatalities from 1950 to 2011. Natural hazards, 71(1), 203-227.
- Park, D., Lee, S., Nikhil, N. V., Kang, S., & Park, J. (2013). Debris flow hazard zonation by probabilistic analysis (Mt. Woomyeon, Seoul, Korea). International Journal of Innovative Research in Science, Engineering and Technology, 2(6), 2381-2390.
- Fuchs, S., Kaitna, R., Scheidl, C., & Hübl, J. (2008). The application of the risk concept to debris flow hazards. Geomechanik und Tunnelbau: Geomechanik und Tunnelbau, 1(2), 120-129.
- Uitto, J. I. (1998). The geography of disaster vulnerability in megacities: A theoretical framework. Applied geography, 18(1), 7-16.
- Liu, Jinfeng, Kana Nakatani, and Takahisa Mizu Yama. "Effect assessment of debris flow mitigation work based on numerical simulation by using Kanako 2D." Landslide, 2013.
- Sung, C. H., & Liaw, S. C. (2020). A GIS-based approach for assessing social vulnerability to flood and debris flow hazards. International journal of disaster risk reduction, 46, 101531.
- Takahashi, T. (2009). A review of Japanese debris flow research. International Journal of Erosion Control Engineering, 2(1), 1-14.
- Takahashi, T. (2009). A review of Japanese debris flow research. International Journal of Erosion Control Engineering, 2(1), 1-14.
- Nettleton, I. M., Martin, S., Hencher, S., & Moore, R. (2005). Debris flow types and mechanisms. Scottish road network landslides study, 45-67.
- PDMA (2016). Overview of disaster in Khyber Pakhtukhawa; Impact respose and managing risk . Provincial Disaster Managment Authority,Khyber Paktunkhwa, Pakistan.
- Blistanova, M., Zeleňáková, M., Blistan, P., & Ferencz, V. (2016). Assessment of flood vulnerability in Bodva river basin, Slovakia. Acta Montanistica Slovaca, 21(1).
- Butt, M. J., Umar, M., & Qamar, R. (2013). Landslide dam and subsequent dam-break flood estimation using HEC-RAS model in Northern Pakistan. Natural Hazards, 65(1), 241-254.
- Coe, J., Cannon, S., & Santi, P. (2008). Debris flows initiated by runoff, erosion, and sediment entrainment in western North America. Geomorphology (Amsterdam), 96(3-4).
- Kogelnig, A., Hübl, J., Suriñach, E., Vilajosana, I., & McArdell, B. W. (2014). Infrasound produced by debris flow: propagation and frequency content evolution. Natural hazards, 70(3), 1713-1733.
- Lan, H. X., Li, L., Zhang, Y. S., Gao, X., & Liu, H. J. (2013). Risk assessment of debris flow in Yushu seismic area in China: a perspective for the reconstruction. Natural Hazards and Earth System Sciences, 13(11), 2957-2968.
- Liu, G., Dai, E., Ge, Q., Wu, W., & Xu, X. (2013). A similarity-based quantitative model for assessing regional debris-flow hazard. Natural hazards, 69(1), 295-310.
- Jakob, M., Holm, K., & McDougall, S. (2016). Debris-flow risk assessment. In Oxford Research Encyclopedia of Natural Hazard Science.
- Staley, D. M., Kean, J. W., Cannon, S. H., Schmidt, K. M., & Laber, J. L. (2013). Objective definition of rainfall intensity–duration thresholds for the initiation of post-fire debris flows in southern California. Landslides, 10(5), 547-562.
- Tang, C., Zhu, J., Ding, J., Cui, X. F., Chen, L., & Zhang, J. S. (2011). Catastrophic debris flows triggered by a 14 August 2010 rainfall at the epicenter of the Wenchuan earthquake. Landslides, 8(4), 485-497.
- Khan, M. A., Haneef, M., Khan, A. S., & Tahirkheli, T. (2013). Debris-flow hazards on tributary junction fans, Chitral, Hindu Kush Range, northern Pakistan. Journal of Asian Earth Sciences, 62, 720-733.
- Uddin, Z., Zaman, T., Anjum, M., Uddin, S., & Uddin, N. (2020). Debris flow mitigation in Chitral Region, Pakistan. In Селевые потоки: катастрофы, риск, прогноз, защита (pp. 282-289).
- Amin, G., Bano, D., Wali, S., & Shah, S. (2020). Comprehensive analysis of surface characteristics of debris flow fans in Gilgit-Baltistan and Chitral regions of Pakistan using remote sensing. In Селевые потоки: катастрофы, риск, прогноз, защита (pp. 122-133).
- Uddin, Z., Anjum, M., Uddin, S., & Uddin, N. (2020). Debris flow hazard of glacial lake in Chitral, Pakistan. In Селевые потоки: катастрофы, риск, прогноз, защита (pp. 272-281).
- Hafeez, S., Ahmed, W., Azam, S., & Khan, S. (2019). Evaluation of landslide hazards at Herth, Chitral, Pakistan. Innovative Infrastructure Solutions, 4(1), 1-10.
- Alamgeer Hussain, D., Shah, B. H., Nayani, K., Wolf, H., Janay, S., & Abid, M. Integrated Watershed Management Plan (IWMP) for Powoor Watershed Yarkhoon Valley District Chitral.