Article
Revisiting immunology textbooks: Considering potential insights based on the role of RNA-guided antiviral defense
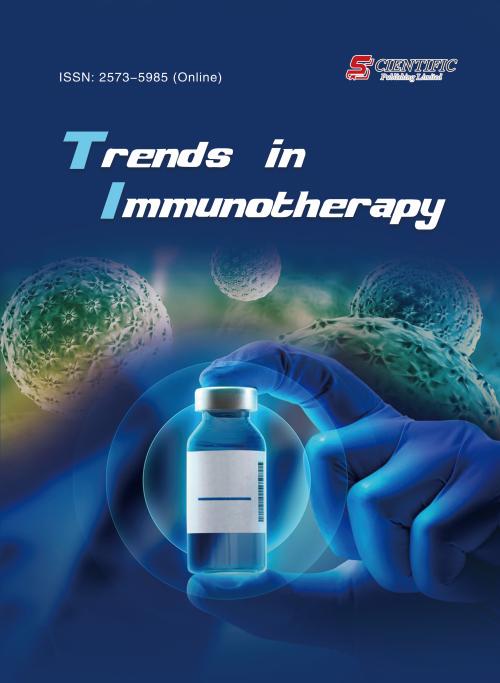
Downloads
Download

This work is licensed under a Creative Commons Attribution 4.0 International License.
Copyright
The authors shall retain the copyright of their work but allow the Publisher to publish, copy, distribute, and convey the work.
License
Trends in Immunotherapy (TI) publishes accepted manuscripts under Creative Commons Attribution 4.0 International (CC BY 4.0). Authors who submit their papers for publication by TI agree to have the CC BY 4.0 license applied to their work, and that anyone is allowed to reuse the article or part of it free of charge for any purpose, including commercial use. As long as the author and original source are properly cited, anyone may copy, redistribute, reuse, and transform the content.
Amidst the COVID-19 pandemic, this article offers a cautious re-evaluation of traditional notions surrounding the immune system’s role in combating viral infections. Departing from the prevalent emphasis on antibodies and T cells, the manuscript proposes a hypothesis highlighting the pivotal role of RNA interference present in every nucleated cell of the human body for antiviral defense. This system, honed over years of co-evolution with viruses, harbors a diverse array of microRNAs aimed at suppressing viral replication. Additionally, in the event of failure, novel microRNAs are synthesized to target specific viruses, underscoring the concept of natural immunity and RNA interference’s adaptability. Delving into the sentinel role of the specialized immune system (SIS), the article underscores its significance in safeguarding the body’s integrity and combating tumors, extending beyond mere adaptation to pathogens. It also discusses the risks associated with antibody-dependent enhancement of infection and the depletion of naive T and B cells resulting from extensive use of antiviral vaccines. By accentuating the significance of RNA interference as an often-overlooked aspect of human antiviral defenses, the authors advocate for broader, more exploratory discussions within the scientific community regarding the intricate nature of immune responses and vaccine efficacy.
Keywords:
antiviral immunity RNA interference CRISPR-Cas interferon antibody-dependent enhancement natural immunity specialized immune systemReferences
- Flajnik MF. A cold-blooded view of adaptive immunity. Nature Reviews Immunology. 2018; 18(7): 438-453. doi: 10.1038/s41577-018-0003-9
- Hirano M, Das S, Guo P, et al. The Evolution of Adaptive Immunity in Vertebrates. Advances in Immunology. 2011; 125-157. doi: 10.1016/b978-0-12-387664-5.00004-2
- Burgess DJ. A body-wide view of somatic mutations. Nature Reviews Genetics. 2021; 22(11): 689-689. doi: 10.1038/s41576-021-00420-1
- Evans EJ, DeGregori J. Cells with cancer-associated mutations overtake our tissues as we age. Aging and Cancer. 2021; 2(3): 82-97. doi: 10.1002/aac2.12037
- Hawkes RA. Enhancement of the infectivity of arboviruses by specific antisera produced in domestic fowls. Australian Journal of Experimental Biology and Medical Science. 1964; 42: 465-482.
- Smatti MK, Al Thani AA, Yassine HM. Viral-Induced Enhanced Disease Illness. Frontiers in Microbiology. 2018; 9. doi: 10.3389/fmicb.2018.02991
- Tirado SMC, Yoon KJ. Antibody-Dependent Enhancement of Virus Infection and Disease. Viral Immunology. 2003; 16(1): 69-86. doi: 10.1089/088282403763635465
- Khandia R, Munjal A, Dhama K, et al. Modulation of Dengue/Zika Virus Pathogenicity by Antibody-Dependent Enhancement and Strategies to Protect Against Enhancement in Zika Virus Infection. Frontiers in Immunology. 2018; 9. doi: 10.3389/fimmu.2018.00597
- Wan Y, Shang J, Sun S, et al. Molecular Mechanism for Antibody-Dependent Enhancement of Coronavirus Entry. Gallagher T, ed. Journal of Virology. 2020; 94(5). doi: 10.1128/jvi.02015-19
- Yip MS, Cheung CY, Li PH, et al. Investigation of Antibody-Dependent Enhancement (ADE) of SARS coronavirus infection and its role in pathogenesis of SARS. BMC Proceedings. 2011; 5(S1). doi: 10.1186/1753-6561-5-s1-p80
- Liu Y, Soh WT, Kishikawa J ichi, et al. An infectivity-enhancing site on the SARS-CoV-2 spike protein targeted by antibodies. Cell. 2021; 184(13): 3452-3466.e18. doi: 10.1016/j.cell.2021.05.032
- Dan JM, Mateus J, Kato Y, et al. Immunological memory to SARS-CoV-2 assessed for up to 8 months after infection. Science. 2021; 371(6529). doi: 10.1126/science.abf4063
- Röltgen K, Powell AE, Wirz OF, et al. Defining the features and duration of antibody responses to SARS-CoV-2 infection associated with disease severity and outcome. Science Immunology. 2020; 5(54). doi: 10.1126/sciimmunol.abe0240
- Wu J, Liang B, Chen C, et al. SARS-CoV-2 infection induces sustained humoral immune responses in convalescent patients following symptomatic COVID-19. Nature Communications. 2021; 12(1). doi: 10.1038/s41467-021-22034-1
- Lapuente D, Winkler TH, Tenbusch M. B-cell and antibody responses to SARS-CoV-2: infection, vaccination, and hybrid immunity. Cellular & Molecular Immunology. 2023; 21(2): 144-158. doi: 10.1038/s41423-023-01095-w
- Chen X, Pan Z, Yue S, et al. Disease severity dictates SARS-CoV-2-specific neutralizing antibody responses in COVID-19. Signal Transduction and Targeted Therapy. 2020; 5(1). doi: 10.1038/s41392-020-00301-9
- Yan J, Bangalore CR, Nikouyan N, et al. Distinct roles of vaccine-induced SARS-CoV-2-specific neutralizing antibodies and T cells in protection and disease. Molecular Therapy. 2024; 32(2): 540-555. doi: 10.1016/j.ymthe.2024.01.007
- Mead MN, Seneff S, Wolfinger R, et al. COVID-19 mRNA Vaccines: Lessons Learned from the Registrational Trials and Global Vaccination Campaign. Cureus; 2024. doi: 10.7759/cureus.52876
- Benson SD, Bamford JKH, Bamford DH, et al. Does Common Architecture Reveal a Viral Lineage Spanning All Three Domains of Life? Molecular Cell. 2004; 16(5): 673-685. doi: 10.1016/j.molcel.2004.11.016
- Krupovič M, Bamford DH. Virus evolution: how far does the double β-barrel viral lineage extend? Nature Reviews Microbiology. 2008; 6(12): 941-948. doi: 10.1038/nrmicro2033
- Nasir A, Caetano-Anollés G. A phylogenomic data-driven exploration of viral origins and evolution. Science Advances. 2015; 1(8). doi: 10.1126/sciadv.1500527
- Knoll AH, Javaux EJ, Hewitt D, et al. Eukaryotic organisms in Proterozoic oceans. Philosophical Transactions of the Royal Society B: Biological Sciences. 2006; 361(1470): 1023-1038. doi: 10.1098/rstb.2006.1843
- Fedonkin MA. The origin of the Metazoa in the light of the Proterozoic fossil record. Paleontological Research. 2003; 7(1): 9-41. doi: 10.2517/prpsj.7.9
- Barrangou R. The roles of CRISPR–Cas systems in adaptive immunity and beyond. Current Opinion in Immunology. 2015; 32: 36-41. doi: 10.1016/j.coi.2014.12.008
- Koonin EV, Makarova KS. Mobile Genetic Elements and Evolution of CRISPR-Cas Systems: All the Way There and Back. Genome Biology and Evolution. 2017; 9(10): 2812-2825. doi: 10.1093/gbe/evx192
- Rosing MT. 13 C-Depleted Carbon Microparticles in > 3700-Ma Sea-Floor Sedimentary Rocks from West Greenland. Science. 1999; 283(5402): 674-676. doi: 10.1126/science.283.5402.674
- Dodd MS, Papineau D, Grenne T, et al. Evidence for early life in Earth’s oldest hydrothermal vent precipitates. Nature. 2017; 543(7643): 60-64. doi: 10.1038/nature21377
- Lee RC, Feinbaum RL, Ambros V. The C. elegans heterochronic gene lin-4 encodes small RNAs with antisense complementarity to lin-14. Cell. 1993; 75(5): 843-854. doi: 10.1016/0092-8674(93)90529-y
- Fire A, Xu S, Montgomery MK, et al. Potent and specific genetic interference by double-stranded RNA in Caenorhabditis elegans. Nature. 1998; 391(6669): 806-811. doi: 10.1038/35888
- Bitko V, Barik S. Phenotypic silencing of cytoplasmic genes using sequence-specific double-stranded short interfering RNA and its application in the reverse genetics of wild type negative-strand RNA viruses. BMC Microbiol. 2001; 1(1): 34. doi: 10.1186/1471-2180-1-34
- Coburn GA, Cullen BR. Potent and Specific Inhibition of Human Immunodeficiency Virus Type 1 Replication by RNA Interference. Journal of Virology. 2002; 76(18): 9225-9231. doi: 10.1128/jvi.76.18.9225-9231.2002
- McCaffrey AP, Nakai H, Pandey K, et al. Inhibition of hepatitis B virus in mice by RNA interference. Nature Biotechnology. 2003; 21(6): 639-644. doi: 10.1038/nbt824
- Yokota T, Sakamoto N, Enomoto N, et al. Inhibition of intracellular hepatitis C virus replication by synthetic and vector-derived small interfering RNAs. EMBO reports. 2003; 4(6): 602-608. doi: 10.1038/sj
- Krönke J, Kittler R, Buchholz F, et al. Alternative Approaches for Efficient Inhibition of Hepatitis C Virus RNA Replication by Small Interfering RNAs. Journal of Virology. 2004; 78(7): 3436-3446. doi: 10.1128/jvi.78.7.3436-3446.2004
- Ge Q, McManus MT, Nguyen T, et al. RNA interference of influenza virus production by directly targeting mRNA for degradation and indirectly inhibiting all viral RNA transcription. Proceedings of the National Academy of Sciences. 2003; 100(5): 2718-2723. doi: 10.1073/pnas.0437841100
- He ML. Inhibition of SARS-Associated Coronavirus Infection and Replication by RNA Interference. JAMA: The Journal of the American Medical Association. 2003; 290(20): 2665-2666. doi: 10.1001/jama.290.20.2665
- Fujino K, Horie M, Honda T, et al. Inhibition of Borna disease virus replication by an endogenous bornavirus-like element in the ground squirrel genome. Proceedings of the National Academy of Sciences. 2014; 111(36): 13175-13180. doi: 10.1073/pnas.1407046111
- Honda T, Tomonaga K. Endogenous non-retroviral RNA virus elements evidence a novel type of antiviral immunity. Mobile Genetic Elements. 2016; 6(3): e1165785. doi: 10.1080/2159256x.2016.1165785
- Watanabe Y, Kishi A, Yachie N, et al. Computational analysis of microRNA‐mediated antiviral defense in humans. FEBS Letters. 2007; 581(24): 4603-4610. doi: 10.1016/j.febslet.2007.08.049
- Jeang KT. RNAi in the regulation of mammalian viral infections. BMC Biology. 2012; 10(1). doi: 10.1186/1741-7007-10-58
- Zhang L, Richards A, Barrasa MI, et al. Reverse-transcribed SARS-CoV-2 RNA can integrate into the genome of cultured human cells and can be expressed in patient-derived tissues. Proceedings of the National Academy of Sciences. 2021; 118(21). doi: 10.1073/pnas.2105968118
- Yin Y, Liu X zhao, He X, et al. Exogenous Coronavirus Interacts with Endogenous Retrotransposon in Human Cells. Frontiers in Cellular and Infection Microbiology. 2021; 11. doi: 10.3389/fcimb.2021.609160
- Liu Y, Rao J, Mi Y, et al. SARS-CoV-2 RNAs are processed into 22-nt vsRNAs in Vero cells. Frontiers in Immunology. 2022; 13. doi: 10.3389/fimmu.2022.1008084
- Dhuli K, Medori MC, Micheletti C, et al. Presence of viral spike protein and vaccinal spike protein in the blood serum of patients with long-COVID syndrome. European Review Medicine Pharmocol Science. 2023; 27(6): 13-19. doi: 10.26355/eurrev_202312_34685
- Aldén M, Olofsson Falla F, Yang D, et al. Intracellular Reverse Transcription of Pfizer BioNTech COVID-19 mRNA Vaccine BNT162b2 In Vitro in Human Liver Cell Line. Current Issues in Molecular Biology. 2022; 44(3): 1115-1126. doi: 10.3390/cimb44030073
- Benmoussa A, Provost P. Milk MicroRNAs in Health and Disease. Comprehensive Reviews in Food Science and Food Safety. 2019; 18(3): 703-722. doi: 10.1111/1541-4337.12424
- Manca S, Upadhyaya B, Mutai E, et al. Milk exosomes are bioavailable and distinct microRNA cargos have unique tissue distribution patterns. Scientific Reports. 2018; 8(1). doi: 10.1038/s41598-018-29780-1
- Zempleni J. Milk exosomes: beyond dietary microRNAs. Genes & Nutrition. 2017; 12(1). doi: 10.1186/s12263-017-0562-6
- Pestka S, Langer JA, Zoon KC, et al. Interferons and Their Actions. Annual Review of Biochemistry. 1987; 56(1): 727-777. doi: 10.1146/annurev.bi.56.070187.003455
- Levy DE. Whence Interferon? Variety in the Production of Interferon in Response to Viral Infection. The Journal of Experimental Medicine. 2002; 195(4): F15-F18. doi: 10.1084/jem.20020075
- Wu J, Chen Z. Innate Immune Sensing and Signaling of Cytosolic Nucleic Acids. Annual review of immunology. 2014; 32: 461-488. doi: 10.1146 /annurev-immunol-032713-120156
- Stetson DB, Medzhitov R. Type I Interferons in Host Defense. Immunity. 2006; 25(3): 373-381. doi: 10.1016/j.immuni.2006.08.007