Review
Immunotherapy in cancer: Where we are and what the future brings?
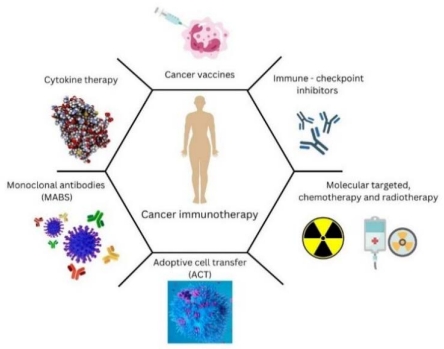
Downloads
Download
Copyright
The authors shall retain the copyright of their work but allow the Publisher to publish, copy, distribute, and convey the work.
License
Trends in Immunotherapy (TI) publishes accepted manuscripts under Creative Commons Attribution 4.0 International (CC BY 4.0). Authors who submit their papers for publication by TI agree to have the CC BY 4.0 license applied to their work, and that anyone is allowed to reuse the article or part of it free of charge for any purpose, including commercial use. As long as the author and original source are properly cited, anyone may copy, redistribute, reuse, and transform the content.
Chemotherapy, radiotherapy, and surgery are recognized as the main treatment modalities for cancer. These therapeutic strategies may be effective in the early stages of the disease but are usually ineffective in advanced stages or when the cancer recurs. Recently, great efforts have been made to understand the complex interaction between the immune system and its surveillance of cancer and to find effective immunotherapies for all stages of cancer. Several types of immunotherapies, including adoptive immunotherapy, cancer vaccines, and immune checkpoint blockades, are receiving considerable attention. The clinical relevance of T lymphocytes and NK cells in combating carcinomas is beyond doubt, but their mechanism of tumor surveillance is far from being fully understood. In this review, much attention has been paid to the complex interplay between T lymphocytes, NK cells, and cancer cells and their role in immunotherapy. Moreover, in this review, we summarized the current data on cancer immunotherapies, especially cancer vaccines, T- and NK cell-based immunotherapies. In addition, we highlighted the role of biomarkers as important indicators of response to immunotherapy, as well as potential problems and solutions related to immunotherapy. Insights into the future of immunotherapy are also presented in this review.
Keywords:
biomarkers cancer immune checkpoint inhibitors immunotherapyReferences
- Wang DR, Wu XL, Sun YL. Therapeutic targets and biomarkers of tumor immunotherapy: Response versus non-response. Signal Transduction Target Therapy 2022; 7(1): 331. doi: 10.1038/s41392-022-01136-2
- Li M, Jiang F, Xue L, et al. Recent progress in biosensors for detection of tumor biomarkers. Molecules 2022; 27(21): 7327. doi: 10.3390/molecules27217327
- Rao Bommi J, Kummari S, Lakavath K, et al. Recent trends in biosensing and diagnostic methods for novel cancer biomarkers. Biosensors (Basel) 2023; 13(3): 398. doi: 10.3390/bios13030398
- Abbott M, Ustoyev Y. Cancer and the immune system: The history and background of immunotherapy. Seminanars in Oncoogyl Nursing 2019; 35(5): 150923. doi: 10.1016/j.soncn.2019.08.002
- Ribatti D. From the discovery of monoclonal antibodies to their therapeutic application: An historical reappraisal. Immunology Letters 2014; 161(1): 96–99. doi: 10.1016/j.imlet.2014.05.010
- Morales A, Eidinger E, Bruce AW. Intracavitary bacillus calmette-guerin in the treatment of superficial bladder tumors. The Journal of Urology 1976; 116(2): 180–182. doi: 10.1016/S0022-5347(17)58737-6
- Dunn GP, Old LJ, Schreiber RD. The immunobiology of cancer immunosurveillance and immunoediting. Immunity 2004; 21(2): 137–148. doi: 10.1016/j.immuni.2004.07.017
- Hanahan D, Weinberg RA. Hallmarks of cancer: The next generation. Cell 2011; 144(5): 646–674. doi: 10.1016/j.cell.2011.02.013
- Yang Y, Lundqvist A. Immunomodulatory effects of IL-2 and IL-15; implications for cancer immunotherapy. Cancers 2020; 12(12): 3586. doi: 10.3390/cancers12123586
- Mansurov A, Lauterbach A, Budina E, et al. Immunoengineering approaches for cytokine therapy. American Journal of Physiology. Cell Physiology 2021; 321(2): C369–C383. doi: 10.1152/ajpcell.00515.2020
- Allison JP. Cd28-B7 interactions in T-cell activation. Current Opinion in Immunology 1994; 6: 414–419. doi: 10.1016/0952-7915(94)90120-1
- Krummel MF, Allison JP. CD28 and CTLA-4 have opposing effects on the response of T-cells to stimulation. Journal of Experimental Mededicine 1995; 182: 459–465. doi: 10.1084/jem.182.2.459
- Hodi FS, Chiarion-Sileni V, Gonzalez R, et al. Nivolumab plus ipilimumab or nivolumab alone versus ipilimumab alone in advanced melanoma (Checkmate 067): 4-year outcomes of a multicentre, randomised, phase 3 trial. Lancet Oncololoy 2018; 19(11): 1480–1492. doi: 10.1016/S1470-2045(18)30700-9
- Ishida Y, Agata Y, Shibahara K, Honjo T. Induced expression of Pd-1, a novel member of the immunoglobulin gene superfamily, upon programmed cell death. The EMBO Journal 1992; 11(11): 3887–3895. doi: 10.1002/j.1460-2075.1992.tb05481.x
- Nishimura H, Nose M, Hiai H, et al. Development of lupus-like autoimmune diseases by disruption of the Pd-1 gene encoding an ITIM motif-carrying immunoreceptor. Immunity 1999; 11(2): 141–151. doi: 10.1016/s1074-7613(00)80089-8
- Dong H, Zhu G, Tamada K, Chen L. B7-H1, a third member of the B7 family, co-stimulates T-cell proliferation and interleukin-10 secretion. Nature Medicine 1999; 5(12): 1365–1369. doi: 10.1038/70932
- Weiden PL, Flournoy N, Thomas ED, et al. Antileukemic effect of graft-versus-host disease in human recipients of allogeneic-marrow grafts. New England Journal of Medicine 1979; 300(19): 1068–1073. doi: 10.1056/NEJM197905103001902
- Gross G, Waks T, Eshhar Z. Expression of immunoglobulin-T-cell receptor chimeric molecules as functional receptors with antibody-type specificity. Proceedings of the National Academy of Sciences of the United States of America 1989; 86(24): 10024–10028. doi: 10.1073/pnas.86.24.10024
- Myers GD, Verneris MR, Goy A, Maziarz RT. Perspectives on outpatient administration of Car-T-cell therapy in aggressive B-cell lymphoma and acute lymphoblastic leukemia. Journal of Immunotherapy Cancer 2021; 9(4): e002056. doi: 10.1136/jitc-2020-002056
- Weber EW, Maus MV, Mackall CL. The emerging landscape of immune cell therapies. Cell 2020; 181(1): 46–62. doi: 10.1016/j.cell.2020.03.001
- Soltantoyeh T, Akbari B, Karimi A, et al. Chimeric antigen receptor (CAR) T-cell therapy for metastatic melanoma: Challenges and road ahead. Cells 2021; 10(6): 1450. doi: 10.3390/cells10061450
- He X, Feng Z, Ma J, et al. Bispecific and split CAR T-cells targeting CD13 and TIM3 eradicate acute myeloid leukemia. Blood 2020; 135(10): 713–723. doi: 10.1182/blood.2019002779
- Duhen R, Fesneau O, Samson KA, et al. PD-1 and ICOS co-expression identifies tumor-reactive CD4 T-cells in human solid tumors. Journal of Clinical Investigations 2022; 132(12): e156821. doi: 10.1172/JCI156821
- Zuo H, Wan Y. Inhibition of myeloid PD-L1 suppresses osteoclastogenesis and cancer bone metastasis. Cancer Gene Therapy 2022; 29(10): 1342–1354. doi: 10.1038/s41417-022-00446-5
- Sanmamed MF, Chen L. A paradigm shift in cancer immunotherapy: From enhancement to normalization. Cell 2018; 175(2): 313–326. doi: 10.1016/j.cell.2018.09.035
- Dersh D, Phelan JD, Gumina ME, et al. Genome-wide screens identify lineage- and tumor-specific genes modulating MHC-I- and MHC-II-restricted immunosurveillance of human lymphomas. Immunity 2021; 54(1): 116–131. doi: 10.1016/j.immuni.2020.11.002
- Hu X, Wang L, Shang B, et al. Immune checkpoint inhibitor-associated toxicity in advanced non-small cell lung cancer: An updated understanding of risk factors. Frontiers in Immunolology 2023; 14: 1094414. doi: 10.3389/fimmu.2023.1094414
- Ghasemi F, Tessier TM, Gameiro SF, et al. High MHC-II expression in Epstein-Barr virus-associated gastric cancers suggests that tumor cells serve an important role in antigen presentation. Science Reports 2020; 10(1): 14786. doi: 10.1038/s41598-020-71775-4
- Burr ML, Sparbier CE, Chan KL, et al. An evolutionarily conserved function of polycomb silences the MHC class I antigen presentation pathway and enables immune evasion in cancer. Cancer Cell 2019; 36(4): 385–401. doi: 10.1016/j.ccell.2019.08.008
- Jalili-Nik M, Soltani A, Mashkani B, et al. PD-1 and PD-L1 inhibitors foster the progression of adult T-cell leukemia/lymphoma. Internal Immunopharmacololoy 2021; 98: 107870. doi: 10.1016/j.intimp.2021.107870
- Wang JY, Doudna JA. CRISPR technology: A decade of genome editing is only the beginning. Science 2023; 379(6629): eadd8643. doi: 10.1126/science.add8643
- Wellhausen N, Agarwal S, Rommel PC, et al. Better living through chemistry: Crispr/Cas engineered T-cells for cancer immunotherapy. Current Opinion in Immunology 2022; 74: 76–84. doi: 10.1016/j.coi.2021.10.008
- Hu Y, Zhou Y, Zhang M, et al. Crispr/Cas9-engineered universal CD19/CD22 dual-targeted CAR-T-cell therapy for relapsed/refractory B-cell acute lymphoblastic leukemia. Clinical Cancer Research 2021; 27(10): 2764–2772. doi: 10.1158/1078-0432.CCR-20-3863
- Lu Y, Xue J, Deng T, et al. Safety and feasibility of CRISPR-edited T-cells in patients with refractory non-small-cell lung cancer. Nature Medicine 2020; 26: 732–740. doi: 10.1038/s41591-020-0840-5
- Agarwal S, Wellhausen N, Levine BL, June CH. Production of human CRISPR-engineered CAR-T-cells. Journal of Visualized Experiments 2021; 15: e62299. doi: 10.3791/62299
- Ai L, Xu A, Xu J. Roles of PD-1/PD-L1 pathway: Signaling, cancer, and beyond. Advances in Experimental Medicine and Biology 2020; 1248: 33–59. doi: 10.1007/978-981-15-3266-5_3
- Sun L, Li CW, Chung EM, et al. Targeting glycosylated PD-1 induces potent antitumor immunity. Cancer Research 2020; 80(11): 2298–2310. doi: 10.1158/0008-5472.CAN-19-3133
- Tang Q, Chen Y, Li X, et al. The role of PD-1/PD-L1 and application of immune-checkpoint inhibitors in human cancers. Frontiers in Immunolology 2022; 13: 964442. doi: 10.3389/fimmu.2022.964442
- Nakamura T, Sato T, Endo R, et al. STING agonist loaded lipid nanoparticles overcome anti-PD-1 resistance in melanoma lung metastasis via NK cell activation. Journal of Immunotherapy Cancer 2021; 9(7): e002852. doi: 10.1136/jitc-2021-002852
- Zhuang Y, Liu C, Liu J, Li G. Resistance mechanism of PD-1/PD-L1 blockade in the cancer-immunity cycle. Oncology Targets Therapy 2020; 13: 83–94. doi: 10.2147/OTT.S239398
- Cao Z, Kon N, Liu Y, et al. An unexpected role for p53 in regulating cancer cell-intrinsic PD-1 by acetylation. Science Advances 2021; 7(14): eabf4148. doi: 10.1126/sciadv.abf4148
- Harjunpää H, Guillerey C. TIGIT as an emerging immune checkpoint. Clinical Experimental Immunolology 2020; 200(2): 108–119. doi: 10.1111/cei.13407
- Sanchez-Correa B, Valhondo I, Hassouneh F, et al. DNAM-1 and the TIGIT/PVRIG/TACTILE axis: Novel immune checkpoints for natural killer cell-based cancer immunotherapy. Cancers 2019; 11(6): 877. doi: 10.3390/cancers11060877
- Levy O, Chan C, Cojocaru G, et al. Abstract 581: Discovery and development of COM701, a therapeutic antibody targeting the novel immune checkpoint PVRIG. Cancer Research 2017; 77(13): 581. doi: 10.1158/1538-7445
- Chu X, Niu L, Xiao G, et al. The long-term and short-term efficacy of immunotherapy in non-small cell lung cancer patients with brain metastases: A systematic review and meta-analysis. Frontiers in Immunolology 2022; 13: 875488. doi: 10.3389/fimmu.2022.875488
- Scheiner B, Roessler D, Phen S, et al. Efficacy and safety of immune checkpoint inhibitor rechallenge in individuals with hepatocellular carcinoma. JHEP Reports 2023; 5(1): 100620. doi: 10.1016/j.jhepr.2022.100620
- Li H, Zhao A, Li M, et al. Targeting T-cell metabolism to boost immune checkpoint inhibitor therapy. Frontiers in Immunology 2022; 13: 1046755. doi: 10.3389/fimmu.2022.1046755
- Ziegler AE, Fittje P, Müller LM, et al. The co-inhibitory receptor TIGIT regulates NK cell function and is upregulated in human intrahepatic CD56(bright) NK cells. Frontiers in Immunology 2023; 14: 1117320. doi: 10.3389/fimmu.2023.1117320
- Banta KL, Xu X, Chitre AS, et al. Mechanistic convergence of the TIGIT and PD-1 inhibitory pathways necessitates co-blockade to optimize anti-tumor CD8+ T-cell responses. Immunity 2022; 55(3): 512–526. doi: 10.1016/j.immuni.2022.02.005
- Cho BC, Abreu DR, Hussein M, et al. Tiragolumab plus atezolizumab versus placebo plus atezolizumab as a first-line treatment for PD-L1-selected non-small-cell lung cancer (CITYSCAPE): Primary and follow-up analyses of a randomised, double-blind, phase 2 study. Lancet Oncology 2022; 23(6): 781–792. doi: 10.1016/S1470-2045(22)00226-1
- Gilfillan S, Chan CJ, Cella M, et al. DNAM-1 promotes activation of cytotoxic lymphocytes by nonprofessional antigen-presenting cells and tumors. Journal of Experimental Medicine 2008; 205(13): 2965–2973. doi: 10.1084/jem.20081752
- Yu X, Harden K, Gonzalez LC, et al. The surface protein TIGIT suppresses T-cell activation by promoting the generation of mature immunoregulatory dendritic cells. Nature Immunolology 2009; 10: 48–57. doi: 10.1038/ni.1674
- Levin SD, Taft DW, Brandt CS, et al. Vstm3 is a member of the CD28 family and an important modulator of T-cell function. European Journal of Immunolology 2011; 41(4): 902–915. doi: 10.1002/eji.201041136
- Ostroumov D, Duong S, Wingerath J, et al. Transcriptome profiling identifies TIGIT as a marker of T-cell exhaustion in liver cancer. Hepatology 2021; 73(4): 1399–1418. doi: 10.1002/hep.31466
- Wherry EJ, Kurachi M. Molecular and cellular insights into T-cell exhaustion. Nature Review Immunolology 2015; 15: 486–499. doi: 10.1038/nri3862
- Mognol GP, Spreafico R, Wong V, et al. Exhaustion-associated regulatory regions in CD8+ tumor-infiltrating T-cells. Proceedings of the National Academy of Sciences of the United States of America 2017; 114(13): E2776–E2785. doi: 10.1073/pnas.1620498114
- Jia B, Zhao C, Rakszawski KL, et al. Eomes+T-betlow CD8+ T-cells are functionally impaired and are associated with poor clinical outcome in patients with acute myeloid leukemia. Cancer Research 2019; 79(7): 1635–1645. doi: 10.1158/0008-5472.CAN-18-3107
- Wang F, Hou H, Wu S, et al. TIGIT expression levels on human NK cells correlate with functional he terogeneity among healthy individuals. European Journal of Immunolology 2015; 45(10): 2886–2897. doi: 10.1002/eji.201545480
- Johnston RJ, Comps-Agrar L, Hackney J, et al. The immunoreceptor TIGIT regulates antitumor and antiviral CD8(+) T-cell effector function. Cancer Cell 2014; 26(6): 923–937. doi: 10.1016/j.ccell.2014.10.018
- Pang K, Shi ZD, Wei LY, et al. Research progress of therapeutic effects and drug resistance of immunotherapy based on PD-1/PD-L1 blockade. Drug Resistance Update 2023; 66: 100907. doi: 10.1016/j.drup.2022.100907
- Zhou K, Li S, Zhao Y, Cheng K. Mechanisms of drug resistance to immune checkpoint inhibitors in non-small cell lung cancer. Frontiers in Immunolology 2023; 14: 1127071. doi: 10.3389/fimmu.2023.1127071
- Liu S, Zhang H, Li M, et al. Recruitment of Grb2 and SHIP1 by the ITT-like motif of TIGIT suppresses granule polarization and cytotoxicity of NK cells. Cell Death and Differentiation 2013; 20(3): 456–464. doi: 10.1038/cdd.2012.141
- Li M, Xia P, Du Y, et al. T-cell immunoglobulin and ITIM domain (TIGIT) receptor/poliovirus receptor (PVR) ligand engagement suppresses interferon-γ production of nat ural killer cells via β-arrestin 2-mediated negative signaling. Journal of Biological Chemistry 2014; 289(25): 17647–17657. doi: 10.1074/jbc.M114.572420
- Stanietsky N, Simic H, Arapovic J, et al. The interaction of TIGIT with PVR and PVRL2 inhibits human NK cell cytotoxicity. Proceedings of the National Academy of Sciences of the United States of America 2009; 106(42): 17858–17863. doi: 10.1073/pnas.0903474106
- Chiang EY, Mellman I. TIGIT-CD226-PVR axis: Advancing immune checkpoint blockade for cancer immunotherapy. Journal of Immunotherapy Cancer 2022; 10(4): e004711. doi: 10.1136/jitc-2022-004711
- Ramsbottom KM, Hawkins ED, Shimoni R, et al. Cutting edge: DNAX accessory molecule 1-deficient CD8+ T-cells display immunological synapse defects that impair antitumor immunity. Journal of Immunology 2014; 192(2): 553–557. doi: 10.4049/jimmunol.1302197
- Shibuya K, Lanier LL, Phillips JH, et al. Physical and functional association of LFA-1 with DNAM-1 adhesion molecule. Immunity 1999; 11(5): 615–623. doi: 10.1016/s1074-7613(00)80136-3
- Du X, de Almeida P, Manieri N, et al. CD226 regulates natural killer cell antitumor responses via phosphorylation-mediated inactivation of transcription factor FOXO1. Proceedings of the National Academy of Sciences of the United States of America 2018; 115(50): E11731–E11740. doi: 10.1073/pnas.1814052115
- Hedrick SM, Hess Michelini R, Doedens AL, et al. FOXO transcription factors throughout T-cell biology. Nature Review of Immunolology 2012; 12(9): 649–661. doi: 10.1038/nri3278
- Deng Y, Kerdiles Y, Chu J, et al. Transcription factor Foxo1 is a negative regulator of natural killer cell maturation and function. Immunity 2015; 42(3): 457–470. doi: 10.1016/j.immuni.2015.02.006
- Shibuya K, Shirakawa J, Kameyama T, et al. CD226 (DNAM-1) is involved in lymphocyte function-associated antigen 1 costimulatory signal for naive T-cell differentiation and proliferation. Journal of Experimental Medicine 2003; 198(12): 1829–1839. doi: 10.1084/jem.20030958
- Diefenbach A, Jamieson AM, Liu SD, et al. Ligands for the murine NKG2D receptor: Expression by tumor cells and activation of NK cells and macrophages. Nature Immunology 2000; 1(2): 119–126. doi: 10.1038/77793
- Kerdiles YM, Stone EL, Beisner DR, et al. Foxo transcription factors control regulatory T-cell development and function. Immunity 2010; 33(6): 890–904. doi: 10.1016/j.immuni.2010.12.002
- Calvet-Mirabent M, Sánchez-Cerrillo I, Martín-Cófreces N, et al. Antiretroviral therapy duration and immunometabolic state determine efficacy of ex vivo dendritic cell-based treatment restoring functional HIV-specific CD8+ T-cells in people living with HIV. EBioMedicine 2022; 81: 104090. doi: 10.1016/j.ebiom.2022.104090
- Fathi M, Bahmanpour S, Barshidi A, et al. Simultaneous blockade of TIGIT and HIF-1α induces synergistic anti-tumor effect and decreases the growth and development of cancer cells. International Immunopharmacolology 2021; 101(Part A): 108288. doi: 10.1016/j.intimp.2021.108288
- de Waal Malefyt R, Haanen J, Spits H, et al. Interleukin 10 (IL-10) and viral IL-10 strongly reduce antigen-specific human T-cell proliferation by diminishing the antigen-presenting capacity of monocytes via downregulation of class II major histocompatibility complex expression. Journal of Experimental Medicine 1991; 174(4): 915–924. doi: 10.1084/jem.174.4.915
- Mao XC, Yang CC, Yang YF, et al. Peripheral cytokine levels as novel predictors of survival in cancer patients treated with immune checkpoint inhibitors: A systematic review and meta-analysis. Frontiers in Immunolology 2022; 13: 884592. doi: 10.3389/fimmu.2022.884592
- Brauneck F, Fischer B, Witt M, et al. TIGIT blockade repolarizes AML-associated TIGIT(+) M2 macrophages to an M1 phenotype and increases CD47-mediated phagocytosis. Journal of Immunotherapy Cancer 2022; 10(12): e004794. doi: 10.1136/jitc-2022-004794
- Mao L, Xiao Y, Yang QC, et al. TIGIT/CD155 blockade enhances anti-PD-L1 therapy in head and neck squamous cell carcinoma by targeting myeloid-derived suppressor cells. Oral Oncology 2021; 121: 105472. doi: 10.1016/j.oraloncology.2021.105472
- Chu X, Tian W, Wang Z, et al. Co-inhibition of TIGIT and PD-1/PD-L1 in cancer immunotherapy: Mechanisms and clinical trials. Molecular Cancer 2023; 22(1): 93. doi: 10.1186/s12943-023-01800-3
- Niu J, Maurice-Dror C, Lee DH, et al. First-in-human phase 1 study of the anti-TIGIT antibody vibostolimab as monotherapy or with pembrolizumab for advanced solid tumors, including non-small-cell lung cancer. Annunal Oncolology 2022; 33(2): 169–180. doi: 10.1016/j.annonc.2021.11.002
- Mettu NB, Ulahannan SV, Bendell JC, et al. A phase 1a/b Open-Label, dose-escalation study of Etigilimab alone or in combination with Nivolumab in patients with locally advanced or metastatic solid tumors. Clinical Cancer Research 2022; 28(5): 882–892. doi: 10.1158/1078-0432.CCR-21-2780
- Zhang J, Hussein M, Kao SC-H, et al. AdvanTIG-105: Phase Ib dose-expansion study of ociperlimab (OCI) + tislelizumab (TIS) with chemotherapy in patients (pts) with extensive-stage small cell lung cancer (ES-SCLC). Immuno-Oncology and Technology 2022; 16: 100260. doi: 10.1016/j.iotech.2022.100260
- Merck Sharp & Dohme LLC. Substudy 02A: Safety and Efficacy of Pembrolizumab in Combination with Investigational Agents in Participants with Programmed Cell-death 1 (PD-1) Refractory Melanoma (MK-3475-02A/KEYMAKER-U02). ClinicalTrials.gov; 2023.
- Sarikonda G, Wallace BK, Wiesner C, et al. 111P interim biomarker analysis of a phase Ib/II study of anti-TIGIT etigilimab (MPH313) and nivolumab in subjects with select locally advan ced or metastatic solid tumors (ACTIVATE). Annual Oncology 2012; 33: S589–S596. doi: 10.1016/j.annonc.2022.07.143
- Ahn IMJ, Cervantes A, Wainberg Z, et al. Phase Ia/Ib dose-escalation study of the anti-TIGIT antibody tiragolumab as a single agent and in combination with atezolizumab in patients with advanced solid tumors. Cancer Research 2020; 80: CT302. doi: 10.1158/1538-7445.AM2020-CT302
- Johnson ML, Fox W, Lee Y-G, et al. ARC-7: Randomized phase 2 study of domvanalimab + zimberelimab ± etrumadenant versus zimberelimab in first-line, metastatic, PD-L1-high non-small cell lung cancer (NSCLC). Journal of Clinical Oncology 2023; 40: 397600. doi: 10.1200/JCO.2022.40.36_suppl.397600
- Rudin CM, Liu SV, Lu S, et al. SKYSCRAPER-02: Primary results of a phase III, randomized, double-blind, placebocontrolled study of atezolizumab (atezo) + carboplatin + etoposide (CE) with or without tiragolumab (tira) in patients (pts) with untreated extensive-stage small cell lung cancer (ES-SCLC). Journal of Clinical Oncology 2022; 40(17): 1–15.
- Grapin M, Richard C, Limagne E, et al. Optimized fractionated radiotherapy with anti-PD-L1 and anti-TIGIT: A promising new combination. Journal of Immunotherapy Cancer 2019; 7(1): 160. doi: 10.1186/s40425-019-0634-9
- Barsoumian HB, Sezen D, Menon H, et al. High plus low dose radiation strategy in combination with TIGIT and PD1 blockade to promote systemic antitumor responses. Cancers 2022; 14(1): 221. doi: 10.3390/cancers14010221
- Zhao K, Jiang L, Si Y, et al. TIGIT blockade enhances tumor response to radiotherapy via a CD103 + dendritic cell-dependent mechanism. Cancer Immunolology Immunotherapy 2023; 72(1): 193–209. doi: 10.1007/s00262-022-03227-z
- Hu Y, Paris S, Bertolet G, et al. Combining a nanoparticle-mediated immunoradiotherapy with dual blockad e of LAG3 and TIGIT improves the treatment efficacy in anti-PD1 resist ant lung cancer. Journal of Nanobiotechnolology 2022; 20(1): 417. doi: 10.1186/s12951-022-01621-4
- Wei SC, Duffy CR, Allison JP. Fundamental mechanisms of immune checkpoint blockade therapy. Cancer Discovery 2018; 8(9): 1069–1086. doi: 10.1158/2159-8290.CD-18-0367
- Grupp SA, Kalos M, Barrett D et al. Chimeric antigen receptor-modified T-cells for acute lymphoid leukemia. The New England journal of medicine 2013; 368(16): 1509–1518. doi: 10.1056/NEJMoa1215134
- Du X, Liu M, Su J, et al. Uncoupling therapeutic from immunotherapy-related adverse effects for safer and effective anti-CTLA-4 antibodies in CTLA4 humanized mice. Cell Research 2018; 28(4): 433–447. doi: 10.1038/s41422-018-0012-z
- Zhang Y, Du X, Liu M, et al. Hijacking antibody-induced CTLA-4 lysosomal degradation for safer and more effective cancer immunotherapy. Cell Research 2019; 29: 609–627. doi: 10.1038/s41422-019-0184-1
- Arce Vargas F, Furness AJS, Litchfield K, et al. Fc effector function contributes to the activity of human anti-CTLA-4 antibodies. Cancer Cell 2018; 33(4): 649–663. doi: 10.1016/j.ccell.2018.02.010
- Du X, Tang F, Liu M, et al. A reappraisal of CTLA-4 checkpoint blockade in cancer immunotherapy. Cell Research 2018; 28(4): 416–432. doi: 10.1038/s41422-018-0011-0
- Cai S, Chen Z, Wang Y, et al. Reducing PD-L1 expression with a self-assembled nanodrug: An alternative to PD-L1 antibody for enhanced chemo-immunotherapy. Theranostics 2021; 11(4): 1970–1981. doi: 10.7150/thno.45777
- Jabbarzadeh Kaboli P, Salimian F, Aghapour S, et al. Akt-targeted therapy as a promising strategy to overcome drug resistance in breast cancer—A comprehensive review from chemotherapy to immunotherapy. Pharmacolology Research 2020; 156: 104806. doi: 10.1016/j.phrs.2020.104806
- Zhang Y, Yue C, Krichevsky AM, Garkavtsev I. Repression of the stress granule protein G3BP2 inhibits immune checkpoint molecule PD-L1. Molecular Oncology 2021. doi: 10.1002/1878-0261.12915
- Liang J, Wang L, Wang C, et al. Verteporfin inhibits PD-L1 through autophagy and the STAT1-IRF1-TRIM28 signaling axis, exerting antitumor efficacy. Cancer Immunology Research 2020; 8(7): 952–965. doi: 10.1158/2326-6066.CIR-19-0159
- Larkin J, Chiarion-Sileni V, Gonzalez R, et al. Five-year survival with combined nivolumab and ipilimumab in advanced melanoma. New England Journal of Medicine 2019; 381(16): 1535–1546. doi: 10.1056/NEJMoa1910836
- Tawbi HA, Forsyth PA, Algazi A, et al. Combined nivolumab and ipilimumab in melanoma metastatic to the Brain. New England Journal of Medicine 2018; 379(8): 722–730. doi: 10.1056/NEJMoa1805453
- Hellmann MD, Ciuleanu TE, Pluzanski A, et al. Nivolumab plus ipilimumab in lung cancer with a high tumor mutational burden. New England Journal of Medicine 2019; 378(22): 2093–2104. doi: 10.1056/NEJMoa1801946
- Motzer RJ, Tannir NM, McDermott DF, et al. Nivolumab plus ipilimumab versus sunitinib in advanced renal-cell carcinoma. New England Journal of Medicine 2018; 378(14): 1277–1290. doi: 10.1056/NEJMoa1712126
- Blank CU, Rozeman EA, Fanchi LF, et al. Neoadjuvant versus adjuvant ipilimumab plus nivolumab in macroscopic stage III melanoma. Nature Medicine 2018; 24(11): 1655–1661. doi: 10.1038/s41591-018-0198-0
- Amaria RN, Reddy SM, Tawbi HA, et al. Neoadjuvant immune checkpoint blockade in high-risk resectable melanoma. Nature Medicine 2018; 24(11): 1649–1654. doi: 10.1038/s41591-018-0197-1
- Liu Y, Zheng P. Preserving the CTLA-4 checkpoint for safer and more effective cancer immunotherapy. Trends in Pharmacolology Science 2020; 41(1): 4–12. doi: 10.1016/j.tips.2019.11.003
- Zhang H, Dai Z, Wu W, et al. Regulatory mechanisms of immune checkpoints PD-L1 and CTLA-4 in cancer. Journal of Experimental Clinical Cancer Research 2021; 40: 184. doi: 10.1186/s13046-021-01987-7
- Shi AP, Tang XY, Xiong YL, et al. Immune checkpoint LAG3 and its ligand FGL1 in cancer. Frontiers in Immunolology 2021; 12: 785091. doi: 10.3389/fimmu.2021.785091
- Yi M, Zheng X, Niu M, et al. Combination strategies with PD-1/PD-L1 blockade: Current advances and future directions. Molecular Cancer 2022; 21(1): 28. doi: 10.1186/s12943-021-01489-2
- Maeda TK, Sugiura D, Okazaki IM, et al. Atypical motifs in the cytoplasmic region of the inhibitory immune co-receptor LAG-3 inhibit T-cell activation. Journal of Biological Chemistry 2019; 294(15): 6017–6026. doi: 10.1074/jbc.RA119.007455
- Andrews LP, Yano H. Vignali DAA. Inhibitory receptors and ligands beyond PD-1, PD-L1 and CTLA-4: Breakthroughs or backups. Nature Immunology 2019; 20: 1425–1434. doi: 10.1038/s41590-019-0512-0
- Lecocq Q, Keyaerts M, Devoogdt N, et al. The next-generation immune checkpoint LAG-3 and its therapeutic potential in oncology: Third time’s a charm. Internal Journal of Molecular Science 2020; 22(1): 75. doi: 10.3390/ijms22010075
- Maruhashi T, Sugiura D, Okazaki IM, et al. LAG-3: From molecular functions to clinical applications. Journal of Immunotherapy Cancer 2020; 8(2): e001014. doi: 10.1136/jitc-2020-001014
- Tawbi HA, Schadendorf D, Lipson EJ, et al. Relatlimab and nivolumab versus nivolumab in untreated advanced melanoma. New England Journal of Medicine 2022; 386: 24–34. doi: 10.1056/NEJMoa2109970
- Maruhashi T, Sugiura D, Okazaki IM, et al. Binding of LAG-3 to stable peptide-MHC class II limits T-cell function and suppresses autoimmunity and anti-cancer immunity. Immunity 2022; 55(5): 912–924. doi: 10.1016/j.immuni.2022.03.013
- Chocarro L, Blanco E, Zuazo M, et al. Understanding LAG-3 signaling. International Journal of Molecular Sciences 2021; 22(10): 5282. doi: 10.3390/ijms22105282
- Chen J, López-Moyado IF, Seo H, et al. Nr4A transcription factors limit CAR T-cell function in solid tumours. Nature 2019; 567: 530–534. doi: 10.1038/s41586-019-0985-x
- Khan O, Giles JR, McDonald S, et al. TOX transcriptionally and epigenetically programs CD8+ T-cell exhaustion. Nature 2019; 571: 211–218. doi: 10.1038/s41586-019-1325-x
- Liu X, Wang Y, Lu H, et al. Genome-wide analysis identifies NR4A1 as a key mediator of T-cell dysfunction. Nature 2019; 567: 525–529. doi: 10.1038/s41586-019-0979-8
- Seo H, Chen J, González-Avalos E, et al. TOX and TOX2 transcription factors cooperate with NR4A transcription factors to impose CD8+ T-cell exhaustion. Proceedings of the National Academy of Sciences of the United States of America 2019; 116(25): 12410–12415. doi: 10.1073/pnas.1905675116
- Scott AC, Dündar F, Zumbo P, et al. Tox is a critical regulator of tumour-specific T-cell differentiation. Nature 2019; 571: 270–274. doi: 10.1038/s41586-019-1324-y
- Rudd CE, Chanthong K, Taylor A. Small molecule inhibition of GSK-3 specifically inhibits the transcription of inhibitory co-receptor LAG-3 for enhanced anti-tumor immunity. Cell Reports 2020; 30(7): 2075–2082. doi: 10.1016/j.celrep.2020.01.076
- Tian D, Yang L, Wang S, et al. Double negative T-cells mediate Lag3-dependent antigen-specific protection in allergic asthma. Nature Community 2019; 10: 4246. doi: 10.1038/s41467-019-12243-0
- Huo JL, Wang YT, Fu WJ, et al. The promising immune checkpoint LAG-3 in cancer immunotherapy: From basic research to clinical application. Front in Immunolology 2022; 13: 956090. doi: 10.3389/fimmu.2022.956090
- Melaiu O, Lucarini V, Giovannoni R, et al. News on immune checkpoint inhibitors as immunotherapy strategies in adult and pediatric solid tumors. Seminars in Cancer Biolology 2022; 79: 18–43. doi: 10.1016/j.semcancer.2020.07.001
- Bagchi S, Yuan R, Engleman EG. Immune checkpoint inhibitors for the treatment of cancer: Clinical impact and mechanisms of response and resistance. Annual Review Patholology 2022; 16: 223–249. doi: 10.1146/annurev-pathol-042020-042741
- Wang J, Sanmamed MF, Datar I, et al. Fibrinogen-like protein 1 is a major immune inhibitory ligand of LAG-3. Cell 2019; 176(1–2): 334–347. doi: 10.1016/j.cell.2018.11.010
- Lecocq Q, Awad RM, De Vlaeminck Y, et al. Single-domain antibody nuclear imaging allows noninvasive quantification of LAG-3 expression by tumor-infiltrating leukocytes and predicts response of immune checkpoint blockade. Journal of Nuclear Medicine 2021; 62(11): 1638–1644. doi: 10.2967/jnumed.120.258871
- Sordo-Bahamonde C, Lorenzo-Herrero S, Gonzalez-Rodriguez AP, et al. LAG-3 blockade with relatlimab (BMS-986016) restores anti-leukemic responses in chronic lymphocytic leukemia. Cancers (Basel) 2021; 13(9): 2112. doi: 10.3390/cancers13092112
- Yu X, Huang X, Chen X, et al. Characterization of a novel anti-human lymphocyte activation gene 3 (LAG-3) antibody for cancer immunotherapy. MAbs 2019; 11(6): 1139–1148. doi: 10.1080/19420862.2019.1629239
- Anderson AC, Joller N, Kuchroo VK. Lag-3, tim-3, and TIGIT: Co-inhibitory receptors with specialized functions in immune regulation. Immunity 2016; 44(5): 989–1004. doi: 10.1016/j.immuni.2016.05.001
- Waldman AD, Fritz JM, Lenardo MJ. A guide to cancer immunotherapy: From T-cell basic science to clinical practice. Nature Reviews Immunology 2020; 20(11): 651–668. doi: 10.1038/s41577-020-0306-5
- Esfahani K, Roudaia L, Buhlaiga N, et al. A review of cancer immunotherapy: From the past, to the present, to the future. Current Oncology 2020; 27(2): S87–S97. doi: 10.3747/co.27.5223
- Hegde P, Chen DS. Top 10 challenges in cancer immunotherapy. Immunity 2020; 52(1): 17–35. doi: 10.1016/j.immuni.2019.12.011
- Igarashi Y, Sasada T. Cancer vaccines: Toward the next breakthrough in cancer immunotherapy. Journal of Immunolology Research 2020; 2020: 5825401. doi: 10.1155/2020/5825401
- Fucikova J, Reddy SM, Tawbi HA, et al. An autologous dendritic cell vaccine promotes anticancer immunity in patients with ovarian cancer with low mutational burden and cold tumors. Clinical Cancer Research 2022; 28(14): 3053–3065. doi: 10.1158/1078-0432.CCR-21-4413
- Li K, Tandurella JA, Gai J, et al. Multi-omic analyses of changes in the tumor microenvironment of pancreatic adenocarcinoma following neoadjuvant treatment with anti-PD-1 therapy. Cancer Cell 2022; 40(11): 1374–1391. doi: 10.1016/j.ccell.2022.10.001
- Basirat E, Dehghan D, Abbasi A, Pakravan N. In vitro and in vivo evidence on intra-tumor injection of allogeneic serum for immunotherapy in a mouse model of colon cancer. Iran Journal of Allergy Asthma Immunolology 2022; 21(5): 549–560. doi: 10.18502/ijaai.v21i5.11042
- Shang S, Zhao Y, Qian K, et al. The role of neoantigens in tumor immunotherapy. Biomedicine & Pharmacotherapy 2022; 151: 113118. doi: 10.1016/j.biopha.2022.113118
- Wells DK, van Buuren MM, Dang KK, et al. Key parameters of tumor epitope immunogenicity revealed through a consortium approach improve neoantigen prediction. Cell 2020; 183(3): 818–834. doi: 10.1016/j.cell.2020.09.015
- Kim S, Kim HS, Kim E, et al. Neopepsee: Accurate genome-level prediction of neoantigens by harnessing sequence and amino acid immunogenicity information. Annuanl Oncolology 2018; 29(4): 1030–1036. doi: 10.1093/annonc/mdy022
- Schenck RO, Lakatos E, Gatenbee C, et al. NeoPredPipe: High-throughput neoantigen prediction and recognition potential pipeline. BMC Bioinformatics 2019; 20(1): 264. doi: 10.1186/s12859-019-2876-4
- Hu Z, Leet DE, Allesoe RL, et al. Personal neoantigen vaccines induce persistent memory T-cell responses and epitope spreading in patients with melanoma. Nature Medicine 2021; 27: 515–525. doi: 10.1038/s41591-020-01206-4
- Giannakis M, Mu XJ, Shukla SA, et al. Genomic correlates of immune-cell infiltrates in colorectal carcinoma. Cell Reports 2016; 15(4): 857–865. doi: 10.1016/j.celrep.2016.03.075
- Keskin DB, Anandappa AJ, Sun J, et al. Neoantigen vaccine generates intratumoral T-cell responses in phase Ib glioblastoma trial. Nature 2019; 565(7738): 234–239. doi: 10.1038/s41586-018-0792-9
- D’Angelo F, Ceccarelli M, Tala, et al. The molecular landscape of glioma in patients with neurofibromatosis 1. Nature Medicine 2019; 25: 176–187. doi: 10.1038/s41591-018-0263-8
- Xie N, Shen G, Gao W, et al. Neoantigens: Promising targets for cancer therapy. Signal Transduction and Target Therapy 2023; 8(1): 9. doi: 10.1038/s41392-022-01270-x
- Quintana A, Peg V, Prat A, et al. Immune analysis of lymph nodes in relation to the presence or absence of tumor infiltrating lymphocytes in triple-negative breast cancer. European Journal of Cancer 2021; 148: 134–145. doi: 10.1016/j.ejca.2021.01.037
- Dreno B, Thompson JF, Smithers BM, et al. MAGE-A3 immunotherapeutic as adjuvant therapy for patients with resected, MAGE-A3-positive, stage III melanoma (DERMA): A double-blind, randomised, placebo-controlled, phase 3 trial. Lancet Oncolology 2018; 19(7): 916–929. doi: 10.1016/S1470-2045(18)30254-7
- Vansteenkiste JF, Cho BC, Vanakesa T, et al. Efficacy of the MAGE-A3 cancer immunotherapeutic as adjuvant therapy in patients with resected MAGE-A3-positive non-small-cell lung cancer (MAGRIT): A randomised, double-blind, placebo-controlled, phase 3 trial. Lancet Oncolology 2016; 17(6): 822–835. doi: 10.1016/S1470-2045(16)00099-1
- Butts C, Socinski MA, Mitchell PL, et al. Tecemotide (L-BLP25) versus placebo after chemoradiotherapy for stage III non-small-cell lung cancer (START): A randomised, double-blind, phase 3 trial. Lancet Oncology 2014; 15(1): 59–68. doi: 10.1016/S1470-2045(13)70510-2
- Hewitt DB, Nissen N, Hatoum H, et al. A phase 3 randomized clinical trial of chemotherapy with or without algenpantucel-L (hyperacute-pancreas) immunotherapy in subjects with borderline resectable or locally advanced unresectable pancreatic cancer. Annals of Surgery 2022; 275(1): 45–53. doi: 10.1097/SLA.0000000000004669
- Rini BI, Stenzl A, Zdrojowy R, et al. IMA901, a multipeptide cancer vaccine, plus sunitinib versus sunitinib alone, as first-line therapy for advanced or metastatic renal cell carcinoma (IMPRINT): A multicentre, open-label, randomised, controlled, phase 3 trial. Lancet Oncology 2016; 17(11): 1599–1611. doi: 10.1016/S1470-2045(16)30408-9
- Inman S. Rintega (Rindopepimut) misses survival endpoint in phase 3 glioblastoma trial. Available online: https://www.curetoday.com/view/rintega-misses-survival-endpoint-in-phase-3-glioblastoma-trial (accessed on 10 March 2016).
- Liu Z, Lv J, Dang Q, et al. Engineering neoantigen vaccines to improve cancer personalized immunotherapy. International Journal of Biology Science 2022; 18(15): 5607–5623. doi: 10.7150/ijbs.76281
- Dudley ME, Wunderlich JR, Yang JC, et al. Adoptive cell transfer therapy following non-myeloablative but lymphodepleting chemotherapy for the treatment of patients with refractory metastatic melanoma. Journal of Clinical Oncology 2005; 23(10): 2346–2357. doi: 10.1200/JCO.2005.00.240
- Rohaan MW, Borch TH, van den Berg JH, et al. Tumor-infiltrating lymphocyte therapy or ipilimumab in advanced melanoma. New England Journal of Medicine 2022; 387(23): 2113–2125. doi: 10.1056/NEJMoa2210233
- Schuster SJ, Bishop MR, Tam CS, et al. Tisagenlecleucel in adult relapsed or refractory diffuse large B-cell lymphoma. New England Journal of Medicine 2019; 380(1): 45–56. doi: 10.1056/NEJMoa1804980
- Kennedy LB, Salama AKS. A review of cancer immunotherapy toxicity. CA Cancer Journal Clinical 2020; 70(2): 86–104. doi: 10.3322/caac.21596
- Melenhorst JJ, Chen GM, Wang M, et al. Decade-long leukaemia remissions with persistence of Cd4+ CAR T-cells. Nature 2022; 602: 503–509. doi: 10.1038/s41586-021-04390-6
- Qin VM, D’Souza C, Neeson PJ, Zhu JJ. Chimeric antigen receptor beyond CAR-T-cells. Cancers 2021; 13(3): 404. doi: 10.3390/cancers13030404
- Adeel K, Fergusson NJ, Shorr R, et al. Efficacy and safety of CD22 chimeric antigen receptor (CAR) T-cell therapy in patients with B-cell malignancies: A protocol for a systematic review and meta-analysis. Systemic Review 2021; 10: 35. doi: 10.1186/s13643-021-01588-7
- Xue G, Zheng N, Fang J, et al. Adoptive cell therapy with tumor-specific Th9 cells induces viral mimicry to eliminate antigen-loss-variant tumor cells. Cancer Cell 2021; 39(12): 1610–1622. doi: 10.1016/j.ccell.2021.09.011
- Hao Z, Li R, Meng L, et al. Macrophage, the potential key mediator in CAR-T related CRS. Experimental Hematoology and Oncolology 2020; 9: 15. doi: 10.1186/s40164-020-00171-5
- Pan J, Deng B, Ling Z, et al. Ruxolitinib mitigates steroid-refractory CRS during CAR T therapy. Journal of Cellular and Molecular Medicine 2021; 25(2): 1089–1099. doi: 10.1111/jcmm.16176
- Lichtenstein DA, Schischlik F, Shao L, et al. Characterization of HLH-like manifestations as a CRS variant in patients receiving CD22 CAR T-cells. Blood 2021; 138(24): 2469–2484. doi: 10.1182/blood.2021011898
- Schubert ML, Schmitt M, Wang L, et al. Side-effect management of chimeric antigen receptor (CAR) T-cell therapy. Annals in Oncology 2021; 32(1): 34–48. doi: 10.1016/j.annonc.2020.10.478
- Majzner RG, Rietberg SP, Sotillo E, et al. Tuning the antigen density requirement for CAR T-cell activity. Cancer Discovery 2020; 10(5): 702–723. doi: 10.1158/2159-8290.CD-19-0945
- Bedoya MD, Dutoit V, Migliorini D. Allogeneic CAR T-cells: An alternative to overcome challenges of CAR T-cell therapy in glioblastoma. Frontiers in Immunolology 2021; 12: 640082. doi: 10.3389/fimmu.2021.640082
- Edeline J, Houot R, Marabelle A, Alcantara M. CAR-T-cells and bites in solid tumors: Challenges and perspectives. Journal of Hematolology and Oncolology 2021; 14(1): 65. doi: 10.1186/s13045-021-01067-5
- Bashiri DA, Yazdi M, Pockley AG, et al. NK cells armed with chimeric antigen receptors (CAR): Roadblocks to successful development. Cells 2021; 10(12): 3390. doi: 10.3390/cells10123390
- Strati P, Neelapu SS. CAR-T failure: Beyond antigen loss and T-cells. Blood 2021; 137(19): 2567–2568. doi: 10.1182/blood.2020010462
- Baird JH, Frank MJ, Craig J, et al. CD22-directed CAR T-cell therapy induces complete remissions in CD19-directed CAR-refractory large B-cell lymphoma. Blood 2021; 137(17): 2321–2325. doi: 10.1182/blood.2020009432
- Weber EW, Parker KR, Sotillo E, et al. Transient rest restores functionality in exhausted CAR-T-cells through epigenetic remodeling. Science 2021; 372(6537): eaba1786. doi: 10.1126/science.aba1786
- Shao M, Teng X, Guo X, et al. Inhibition of calcium signaling prevents exhaustion and enhances anti-leukemia efficacy of CAR-T-cells via SOCE-calcineurin-NFAT and glycolysis pathways. Advances in Science 2022; 9(9): e2103508. doi: 10.1002/advs.202103508
- Kiesgen S, Messinger JC, Chintala NK, et al. Comparative analysis of assays to measure CAR T-cell-mediated cytotoxicity. Nature Protocols 2021; 16(3): 1331–1342. doi: 10.1038/s41596-020-00467-0
- Marofi F, Al-Awad AS, Rahman HS, et al. CAR-NK cell: A new paradigm in tumor immunotherapy. Froniers in Oncolology 2021; 11: 673276. doi: 10.3389/fonc.2021.673276
- Marofi F, Abdul-Rasheed OF, Rahman HS, et al. CAR-NK cell in cancer immunotherapy; a promising frontier. Cancer Science 2021; 112(9): 3427–3436. doi: 10.1111/cas.14993
- Liu E, Tong Y, Dotti G, et al. Cord blood NK cells engineered to express Il-15 and a CD19-targeted car show long-term persistence and potent antitumor activity. Leukemia 2018; 32(2): 520–531. doi: 10.1038/leu.2017.226
- Myers JA, Miller JS. Exploring the NK cell platform for cancer immunotherapy. Nature Review Clinical Oncology 2021; 18: 85–100. doi: 10.1038/s41571-020-0426-7
- Delgoffe GM, Xu C, Mackall CL, et al. The role of exhaustion in CAR T-cell therapy. Cancer Cell 2021; 39(7): 885–888. doi: 10.1016/j.ccell.2021.06.012
- Tallantyre EC, Evans NA, Parry-Jones J, et al. Neurological updates: Neurological complications of CAR-T therapy. Journal of Neurolology 2021; 268(4): 1544–1554. doi: 10.1007/s00415-020-10237-3
- Poorebrahim M, Melief J, Pico de Coaña Y, et al. Counteracting CAR T-cell dysfunction. Oncogene 2021; 40(2): 421–435. doi: 10.1038/s41388-020-01501-x
- Palucka K, Banchereau J. Dendritic-cell-based therapeutic cancer vaccines. Immunity 2013; 39(1): 38–48. doi: 10.1016/j.immuni.2013.07.004
- Mantovani A, Allavena P, Marchesi F, Garlanda C. Macrophages as tools and targets in cancer therapy. Nature Review of Drugs Discovery 2022; 21(11): 799–820. doi: 10.1038/s41573-022-00520-5
- Zamir MR, Shahi A, Salehi S, Amirzargar A. Natural killer cells and killer cell immunoglobulin-like receptors in solid organ transplantation: Protectors or opponents? Transplantation Review (Orlando) 2022; 36(4): 100723. doi: 10.1016/j.trre.2022.100723
- Zhang Q, Wei W, Wang P, et al. Biomimetic magnetosomes as versatile artificial antigen-presenting cells to potentiate T-cell-based anticancer therapy. ACS Nano 2017; 11(11): 10724–10732. doi: 10.1021/acsnano.7b04955
- Haist H, Mailander V, Bros M. Nanodrugs targeting T-cells in tumor therapy. Frontiers in Immunolology 2022; 13: 912594. doi: 10.3389/fimmu.2022.912594
- Ma X, Li SJ, Liu Y, et al. Bioengineered nanogels for cancer immunotherapy. Chemical Society Review 2022; 51(12): 5136–5174. doi: 10.1039/D2CS00247G
- Raza A, Rossi GR, Janjua TI, et al. Nanobiomaterials to modulate natural killer cell responses for effective cancer immunotherapy. Trends in Biotechnology 2023; 41(1): 77–92. doi: 10.1016/j.tibtech.2022.06.011
- Feng X, Xu W, Li Z, et al. Immunomodulatory nanosystems. Advanced Science 2019; 6(17): 1900101. doi: 10.1002/advs.201900101
- Liu Z, Jiang W, Nam J, et al. Immunomodulating nanomedicine for cancer therapy. Nano Letters 2018; 18(11): 6655–6659. doi: 10.1021/acs.nanolett.8b02340
- Batty CJ, Bachelder EM, Ainslie KM. Historical perspective of clinical nano and microparticle formulations for delivery of therapeutics. Trends in Molecular Medicine 2021; 27(6): 516–519. doi: 10.1016/j.molmed.2021.04.002
- Mangal S, Gao W, Li T, Zhou Q. Pulmonary delivery of nanoparticle chemotherapy for the treatment of lung cancers: Challenges and opportunities. Acta Pharmaceutica Sinica 2017; 38(6): 782–797. doi: 10.1038/aps.2017.34
- Behzadi S, Serpooshan V, Tao W, et al. Cellular uptake of nanoparticles: Journey inside the cell. Chemical Society Reviews 2017; 46(14): 4218–4244. doi: 10.1039/c6cs00636a
- Mitchell MJ, Billingsley MM, Haley RM, et al. Engineering precision nanoparticles for drug delivery. Nature Review of Drug Discovery 2021; 20(2): 101–124. doi: 10.1038/s41573-020-0090-8
- Chen J, Cong X. Surface-engineered nanoparticles in cancer immune response and immunotherapy: Current status and future prospects. Biomedical Pharmacotherapy 2023; 157: 113998. doi: 10.1016/j.biopha.2022.113998
- Mitragotri S, Lammers T, Bae YH, et al. Drug delivery research for the future: Expanding the nano horizons and beyond. Journal od Control Release 2017; 246: 183–184. doi: 10.1016/j.jconrel.2017.01.011
- Gargett T, Abbas MN, Rolan P, et al. Phase I trial of Lipovaxin-MM, a novel dendritic cell-targeted liposomal vaccine for malignant melanoma. Cancer Immunolology and Immunotherapy 2018; 67(9): 1461–1472. doi: 10.1007/s00262-018-2207-z
- Powell E, Chow LQ. BLP-25 liposomal vaccine: A promising potential therapy in non-small-cell lung cancer. Expert Review of Respiratory Medicine 2008; 2(1): 37–45. doi: 10.1586/17476348.2.1.37
- Wurz GT, Gutierrez AM, Greenberg BE, et al. Antitumor effects of L-BLP25 antigen-specific tumor immunotherapy in a novel human MUC1 transgenic lung cancer mouse model. Journal of Translational Medicine 2013; 11: 64. doi: 10.1186/1479-5876-11-64
- Kumthekar P, Ko CH, Paunesku T, et al. A first-in-human phase 0 clinical study of RNA interference-based spherical nucleic acids in patients with recurrent glioblastoma. Science of Translational Medicine 2021; 13(584): eabb3945. doi: 10.1126/scitranslmed.abb3945
- Krpina K, Mavrinac M, Samarzija M, et al. Significance of the lung immune prognostic index for assessment of the reliability of the clinical treatment outcome for advanced non-small-cell lung cancer in patients with COVID-19 infection. Journal of Clinical Medicine 2022; 11(22): 6695. doi: 10.3390/jcm11226695
- Yang SR, Schultheis AM, Yu H, et al. Precision medicine in non-small cell lung cancer: Current applications and future directions. Seminars in Cancer Biology 2022; 84: 184–198. doi: 10.1016/j.semcancer.2020.07.009
- Moutafi M, Martinez-Morilla S, Divakar P, et al. Discovery of biomarkers of resistance to immune checkpoint blockade in NSCLC using high-plex digital spatial profiling. Journal of Thoracic Oncology 2022; 17(8): 991–1001. doi: 10.1016/j.jtho.2022.04.009
- Bhardwaj M, Weigl K, Tikk K, et al. Multiplex screening of 275 plasma protein biomarkers to identify a signature for early detection of colorectal cancer. Molecular Oncology 2020; 14(1): 8–21. doi: 10.1002/1878-0261.12591
- Nixon AB, Sibley AB, Liu Y, et al. Plasma protein biomarkers in advanced or metastatic colorectal cancer patients receiving chemotherapy with bevacizumab or cetuximab: Results from CALGB 80405 (Alliance). Clinical Cancer Research 2022; 28(13): 2779–2788. doi: 10.1158/1078-0432.CCR-21-2389
- Le DT, Kim TW, Van Cutsem E, et al. Phase II open-label study of pembrolizumab in treatment-refractory, microsatellite instability-high/mismatch repair-deficient metastatic colorectal cancer: KEYNOTE-164. Journal of Clinical Oncology 2020; 38(1): 11–19. doi: 10.1200/JCO.19.02107
- Zeng Z, Yang B, Liao Z. Biomarkers in immunotherapy-based precision treatments of digestive system tumors. Frontiers in Oncology 2021; 11: 650481. doi: 10.3389/fonc.2021.650481
- Hou W, Yi C, Zhu H. Predictive biomarkers of colon cancer immunotherapy: Present and future. Frontiers in Immunology 2022; 13: 1032314. doi: 10.3389/fimmu.2022.1032314
- Xu Y, Cao C, Zhu Z, et al. Novel hypoxia-associated gene signature depicts tumor immune microenvironment and predicts prognosis of colon cancer patients. Frontiers Genetics 2022; 13: 901734. doi: 10.3389/fgene.2022.901734
- Jiang M, Qin B, Luo L, et al. A clinically acceptable strategy for sensitizing anti-PD-1 treatment by hypoxia relief. Journal of Controlled Release 2021; 335: 408–419. doi: 10.1016/j.jconrel.2021.06.001
- Ravensbergen CJ, Polack M, Roelands J, et al. Combined assessment of the tumor-stroma ratio and tumor immune cell infiltrate for immune checkpoint inhibitor therapy response prediction in colon cancer. Cells 2021; 10(11): 2935. doi: 10.3390/cells10112935
- Khaliq AM, Erdogan C, Kurt Z, et al. Refining colorectal cancer classification and clinical stratification through a single-cell atlas. Genome Biology 2022; 23(1): 113. doi: 10.1186/s13059-022-02677-z
- Summerfield C, Smith L, Todd O, et al. The effect of older age and frailty on the time to diagnosis of cancer: A connected Bradford electronic health records study. Cancers 2022; 14(22): 5666. doi: 10.3390/cancers14225666
- Whitaker K. Earlier diagnosis: The importance of cancer symptoms. Lancet Oncolology 2020; 21(1): 6–8. doi: 10.1016/S1470-2045(19)30658-8
- Royal RE, Levy C, Turner K, et al. Phase 2 trial of single agent Ipilimumab (anti-CTLA-4) for locally advanced or metastatic pancreatic adenocarcinoma. Journal of Immunotherapy 2010; 33(8): 828–833. doi: 10.1097/CJI.0b013e3181eec14c
- Zhu AX, Finn RS, Edeline J, et al. Pembrolizumab in patients with advanced hepatocellular carcinoma previously treated with sorafenib (KEYNOTE-224): A non-randomised, open-label phase 2 trial. Lancet Oncology 2018; 19(7): 940–952. doi: 10.1016/S1470-2045(18)30351-6
- Eshkiki ZS, Agah S, Tabaeian SP, et al. Neoantigens and their clinical applications in human gastrointestinal cancers. World Journal of Surgical Oncology 2022; 20(1): 321. doi: 10.1186/s12957-022-02776-y
- Veatch JR, Jesernig BL, Kargl J, et al. Endogenous CD4+ T-cells recognize neoantigens in lung cancer patients, including recurrent oncogenic KRAS and ERBB2 (Her2) driver mutations. Cancer Immunology Research 2019; 7(6): 910–922. doi: 10.1158/2326-6066.CIR-18-0402
- Rodriquenz MG, Negrete-Najar JP, Sam C, et al. Assessment of the external validity of the National Comprehensive Cancer Network (NCCN) guidelines for pancreatic ductal adenocarcinoma in a population of older patients aged 70 years and older. Journal of Geriatric Oncology 2022; 13(7): 952–961. doi: 10.1016/j.jgo.2022.04.015
- Wang-Gillam A, Hubner RA, Siveke JT, et al. NAPOLI-1 phase 3 study of liposomal irinotecan in metastatic pancreatic cancer: Final overall survival analysis and characteristics of long-term survivors. European Journal of Cancer 2019; 108: 78–87. doi: 10.1016/j.ejca.2018.12.007
- Mukherji R, Debnath D, Hartley ML, et al. The role of immunotherapy in pancreatic cancer. Current Oncology 2022; 29(10): 6864–6892. doi: 10.3390/curroncol29100541
- Aglietta M, Barone C, Sawyer MB, et al. A phase I dose escalation trial of tremelimumab (CP-675,206) in combination with gemcitabine in chemotherapy-naive patients with metastatic pancreatic cancer. Annals of Oncology 2014; 25(9): 1750–1755. doi: 10.1093/annonc/mdu205
- Kalyan A, Kircher SM, Mohindra NA, et al. Ipilimumab and gemcitabine for advanced pancreas cancer: A phase Ib study. Journal of Clinical Oncology 2016; 34(15): e15747.
- Neelapu SS, Tummala S, Kebriaei P, et al. Chimeric antigen receptor T-cell therapy—Assessment and management of toxicities. Nature Review of Clinical Oncology 2018; 15: 47–62. doi: 10.1038/nrclinonc.2017.148
- Teachey DT, Bishop MR, Maloney DG, Grupp SA. Toxicity management after chimeric antigen receptor T-cell therapy: one size does not fit ‘ALL’. Nature Reviews. Clinical Oncology 2018; 15(4): 218. doi: 10.1038/nrclinonc.2018.19
- Thompson JA. New NCCN guidelines: Recognition and management of immunotherapy-related toxicity. Journal of the National Comprehensive Cancer Network 2018; 16(5): 594–596. doi: 10.6004/jnccn.2018.0047
- Weber JS, Yang JC, Atkins MB, Disis ML. Toxicities of immunotherapy for the practitioner. Journal of Clinical Oncology 2015; 33(18): 2092–2099.
- Postow MA, Sidlow R, Hellmann MD. Immune-related adverse events associated with immune checkpoint blockade. New England Journal of Medicine 2018; 378: 158–168. doi: 10.1056/NEJMra1703481
- Shoushtari AN, Friedman CF, Navid-Azarbaijani P, et al. Measuring toxic effects and time to treatment failure for nivolumab plus ipilimumab in melanoma. JAMA Oncology 2018; 4(1): 98–101. doi: 10.1001/jamaoncol.2017.2391
- Khan U, Rizvi H, Sano D, et al. Nivolumab induced myxedema crisis. Journal of Immunotherapy Cancer 2017; 5: 13. doi: 10.1186/s40425-017-0213-x
- Escudier M, Cautela J, Malissen N, et al. Clinical features, management, and outcomes of immune checkpoint inhibitor-related cardiotoxicity. Circulation 2017; 136: 2085–2087. doi: 10.1161/CIRCULATIONAHA.117.030571
- Naidoo J, Wang X, Woo KM, et al. Pneumonitis in patients treated with anti-programmed death-1/programmed death ligand 1 therapy. Journal of Clinical Oncology 2017; 35(7): 709–717. doi: 10.1200/JCO.2016.68.2005
- Kang K, Wu Y, Yao Z, Lu Y. Tackling the current dilemma of immunotherapy in extensive-stage small cell lung cancer: A promising strategy of combining with radiotherapy. Cancer Letters 2023; 565: 216239. doi: 10.1016/j.canlet.2023.216239
- Babar Q, Saeed A, Tabish TA, et al. Targeting the tumour microenvironment: Potential strategy for cancer therapeutics. Biochimica et Biophysica Acta (BBA)—Molecular Basis of Disease 2023; 1869(6): 166746. doi: 10.1016/j.bbadis.2023.166746
- Guo R, Li J, Hu J, et al. Combination of epidrugs with immune checkpoint inhibitors in cancer immunotherapy: From theory to therapy. Internal Immunopharmacology 2023; 120: 110417. doi: 10.1016/j.intimp.2023.110417
- Dibajnia P, Cardenas LM, Lalani AKA. The emerging landscape of neo/adjuvant immunotherapy in renal cell carcinoma. Human Vaccination Immunotherapy 2023; 19(1): 2178217. doi: 10.1080/21645515.2023.2178217
- von Baumgarten L, Stauss HJ, Lünemann JD. Synthetic cell-based immunotherapies for neurologic diseases. Neurollogy Neuroimmunology Neuroinflammation 2023; 10(5): e200139. doi: 10.1212/NXI.0000000000200139
- Dubbs SB, Falat C, Rosenblatt L. Immune-based therapies—What the emergency physician needs to know. Emergency Medicine Clinics of North America 2023; 40(1): 569–582. doi: 10.1016/j.emc.2021.08.011
- Qin Y, Wang Q, Shi J. Immune checkpoint modulating T-cells and NK cells response to Mycobacterium tuberculosis infection. Microbiology Research 2023; 273: 127393. doi: 10.1016/j.micres.2023.127393
- Wang Q, Shen X, Chen G, Du J. How to overcome resistance to immune checkpoint inhibitors in colorectal cancer: From mechanisms to translation. International Journal of Cancer 2023; 153(4): 709–722. doi: 10.1002/ijc.34464
- Cicala CM, Musacchio L, Scambia G, Lorusso D. Dostarlimab: From preclinical investigation to drug approval and future directions. Human Vaccination Immunotherapy 2023; 19(1): 2178220. doi: 10.1080/21645515.2023.2178220
- Niu H, Zhao P, Sun W. Biomaterials for chimeric antigen receptor T-cell engineering. Acta Biomaterialia 2023; 166: 1–13. doi: 10.1016/j.actbio.2023.04.043
- Najafi S, Mortezaee K. Advances in dendritic cell vaccination therapy of cancer. Biomedicine and Pharmacotherapy 2023; 164: 114954. doi: 10.1016/j.biopha.2023.114954