Review
Revolutionizing cancer treatment by boosting dendritic cell vaccine efficacy with graphene oxide
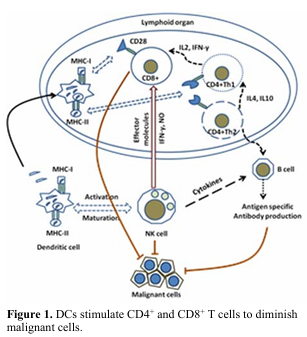
Downloads
Download
Copyright
The authors shall retain the copyright of their work but allow the Publisher to publish, copy, distribute, and convey the work.
License
Trends in Immunotherapy (TI) publishes accepted manuscripts under Creative Commons Attribution 4.0 International (CC BY 4.0). Authors who submit their papers for publication by TI agree to have the CC BY 4.0 license applied to their work, and that anyone is allowed to reuse the article or part of it free of charge for any purpose, including commercial use. As long as the author and original source are properly cited, anyone may copy, redistribute, reuse, and transform the content.
Dendritic cells (DCs) are potent antigen presenting cells that play a crucial role in stimulating T cell responses against cancer. DC vaccines have been utilized as an immunotherapy approach for cancer treatment, but their effectiveness is hampered by challenges in the tumor microenvironment. Graphene oxide (GO), a cutting-edge carbon-based nanomaterial, has shown promise in modulating DC activation and function. This review highlights the recent advancements in DC vaccines and explores how GO can enhance their efficacy for cancer treatment. By leveraging the unique properties of GO, such as its biocompatibility and immunomodulatory effects, DC vaccines can potentially be optimized to overcome the limitations of the tumor microenvironment and achieve improved outcomes in cancer immunotherapy.
Keywords:
Dendritic Cells DC Vaccine Immunotherapy Graphene Oxide CancerReferences
- Wculek SK, Cueto FJ, Mujal AM, et al. Dendritic cells in cancer immunology and immunotherapy. Nature Reviews Immunology 2020; 20(1): 7–24. doi: 10.1038/s41577-019-0210-z.
- Wang Y, Xiang Y, Xin VW, et al. Dendritic cell biology and its role in tumor immunotherapy. Journal of Hematology & Oncology 2020; 13(1): 107. doi: 10.1186/s13045-020-00939-6.
- Yu J, Sun H, Cao W, et al. Research progress on dendritic cell vaccines in cancer immunotherapy. Experimental Hematology & Oncology 2022; 11: 3. doi: 10.1186/s40164-022-00257-2.
- Parente P, Parcesepe P, Covelli C, et al. Crosstalk between the tumor microenvironment and immune system in pancreatic ductal adenocarcinoma: Potential targets for new therapeutic approaches. Gastroenterology Research and Practice 2018; 2018: 7530619. doi: 10.1155/2018/7530619.
- Wang M, Zhao J, Zhang L, et al. Role of tumor microenvironment in tumorigenesis. Journal of Cancer 2017; 8(5): 761–773. doi: 10.7150/jca.17648.
- Chen BJ, Zhao JW, Zhang DH, et al. Immunotherapy of cancer by targeting regulatory T cells. International Immunopharmacology 2022; 104: 108469. doi: 10.1016/j.intimp.2021.108469.
- Malekghasemi S, Majidi J, Baghbanzadeh A, et al. Tumor-associated macrophages: Protumoral macrophages in inflammatory tumor microenvironment. Advanced Pharmaceutical Bulletin 2020; 10(4): 556–565. doi: 10.34172/apb.2020.066.
- Wang Y, Jia A, Bi Y, et al. Targeting myeloid-derived suppressor cells in cancer immunotherapy. Cancers 2020; 12(9): 1–24. doi: 10.3390/cancers12092626.
- Verma A, Mathur R, Farooque A, et al. T-regulatory cells in tumor progression and therapy. Cancer Management and Research 2019; 11: 10731–10747. doi: 10.2147/CMAR.S228887.
- Galli F, Aguilera JV, Palermo B, et al. Relevance of immune cell and tumor microenvironment imaging in the new era of immunotherapy. Journal of Experimental & Clinical Cancer Research 2020; 39: 89. doi: 10.1186/s13046-020-01586-y.
- Farc O, Cristea V. An overview of the tumor microenvironment, from cells to complex networks (Review). Experimental and Therapeutic Medicine 2021; 21(1): 96. doi: 10.3892/etm.2020.9528.
- Labani-Motlagh A, Ashja-Mahdavi M, Loskog A. The tumor microenvironment: A milieu hindering and obstructing antitumor immune responses. Frontiers in Immunology 2020; 11: 940. doi: 10.3389/fimmu.2020.00940.
- Ostroumov D, Fekete-Drimusz N, Saborowski M, et al. CD4 and CD8 T lymphocyte interplay in controlling tumor growth. Cellular and Molecular Life Sciences 2018; 75: 689–713. doi: 10.1007/s00018-017-2686-7.
- Anderson NM, Simon MC. The tumor microenvironment. Currrent Biology 2020; 30(16): R921–R925. doi: 10.1016/j.cub.2020.06.081.
- Binnewies M, Mujal AM, Pollack JL, et al. Unleashing type-2 dendritic cells to drive protective antitumor CD4+ T cell immunity. Cell 2019; 177: 556–571. doi: 10.1016/j.cell.2019.02.005.
- Collin M, Bigley V. Human dendritic cell subsets: An update. Immunology 2018; 154(1): 3–20. doi: 10.1111/imm.12888.
- Gupta YH, Khanom A, Acton SE. Control of dendritic cell function within the tumour microenvironment. Frontiers in Immunology 2022; 13: 733800. doi: 10.3389/fimmu.2022.733800.
- Law AMK, Valdes-Mora F, Gallego-Ortega D. Myeloid-derived suppressor cells as a therapeutic target for cancer. Cells 2020; 9(3): 561. doi: 10.3390/cells9030561.
- Lavin Y, Kobayashi S, Leader A, et al. Innate immune landscape in early lung adenocarcinoma by paired single-cell analyses. Cell 2017; 169(4): 750–765. doi: 10.1016/j.cell.2017.04.014.
- Veglia F, Gabrilovich DI. Dendritic cells in cancer: The role revisited. Current Opinion in Immunology 2017; 45: 43–51. doi: 10.1016/j.coi.2017.01.002.
- Lee JH, Choi SY, Jung NC, et al. The effect of the tumor microenvironment and tumor-derived metabolites on dendritic cell function. Journal of Cancer 2020; 11(4): 769–775. doi: 10.7150/jca.38785.
- Ding J, Zheng Y, Wang G, et al. The performance and perspectives of dendritic cell vaccines modified by immune checkpoint inhibitors or stimulants. Biochimica et Biophysica Acta (BBA)-Reviews on Cancer 2022; 1877(5): 188763. doi: 10.1016/j.bbcan.2022.188763.
- Yu Z, Liu W, He Y, et al. HLA-A2.1-restricted ECM1-derived epitope LA through DC cross-activation priming CD8+ T and NK cells: A novel therapeutic tumour vaccine. Journal of Hematology & Oncology 2021; 14(1): 71. doi: 10.1186/s13045-021-01081-7.
- Najibi AJ, Mooney DJ. Cell and tissue engineering in lymph nodes for cancer immunotherapy. Advanced Drug Delivery Reviews 2020; 161–162: 42–62. doi: 10.1016/j.addr.2020.07.023.
- Galati D, Zanotta S. Hematologic neoplasms: Dendritic cells vaccines in motion. Clinical Immunology 2017; 183:181–190. doi: 10.1016/j.clim.2017.08.016.
- Badiee A, Davies N, McDonald K, et al. Enhanced delivery of immunoliposomes to human dendritic cells by targeting the multilectin receptor DEC-205. Vaccine 2007; 25(25): 4757–4766. doi: 10.1016/j.vaccine.2007.04.029.
- Cauwels A, Van Lint S, Garcin G, et al. A safe and highly efficient tumor-targeted type I interferon immunotherapy depends on the tumor microenvironment. Oncoimmunology 2017; 7(3): e1398876. doi: 10.1080/2162402X.2017.1398876.
- Madan RA, Antonarakis ES, Drake CG, et al. Putting the pieces together: Completing the mechanism of action Jigsaw for Sipuleucel-T. Journal of the National Cancer Institute 2020; 112(6): 562–573. doi: 10.1093/jnci/djaa021.
- Anguille S, Van de Velde AL, Smits EL, et al. Dendritic cell vaccination as postremission treatment to prevent or delay relapse in acute myeloid leukemia. Blood 2017; 130(15): 1713–1721. doi: 10.1182/blood-2017-04-780155.
- Amberger DC, Schmetzer HM. Dendritic cells of leukemic origin: Specialized antigen-presenting cells as potential treatment tools for patients with myeloid leukemia. Transfusion Medicine and Hemotherapy 2020; 47(6): 432–443. doi: 10.1159/000512452.
- Rosenblatt J, Avivi I, Vasir B, et al. Vaccination with dendritic cell/tumor fusions following autologous stem cell transplant induces immunologic and clinical responses in multiple myeloma patients. Clinical Cancer Research 2013; 19(13): 3640–3648. doi: 10.1158/1078-0432.CCR-13-0282.
- Garrido F, Ruiz-Cabello F, Aptsiauri N. Rejection versus escape: The tumor MHC dilemma. Cancer Immunology, Immunotherapy 2017; 66(2): 259–271. doi: 10.1007/s00262-016-1947-x.
- Sun X, Cui Y, Feng H, et al. TGF-β signaling controls Foxp3 methylation and T reg cell differentiation by modulating Uhrf1 activity. Journal of Experimental Medicine 2019; 216(12): 2819–2837. doi: 10.1084/jem.20190550.
- Chen JY, Lai YS, Chu PY, et al. Cancer-derived VEGF-C increases chemokine production in lymphatic endothelial cells to promote CXCR2-dependent cancer invasion and MDSC recruitment. Cancers 2019; 11(8): 1120. doi: 10.3390/cancers11081120.
- Bah I, Kumbhare A, Nguyen L, et al. IL-10 induces an immune repressor pathway in sepsis by promoting S100A9 nuclear localization and MDSC development. Cellular Immunology 2018; 332: 32–38. doi: 10.1016/j.cellimm.2018.07.003.
- Rapp M, Wintergerst MWM, Kunz WG, et al. CCL22 controls immunity by promoting regulatory T cell communication with dendritic cells in lymph nodes. Journal of Experimental Medicine 2019; 216(5): 1170–1181. doi: 10.1084/jem.20170277.
- Burgdorf S, Porubsky S, Marx A, Popovic ZV. Cancer acidity and hypertonicity contribute to dysfunction of tumor-associated dendritic cells: Potential impact on antigen cross-presentation machinery. Cancers 2020; 12(9): 2403. doi: 10.3390/cancers12092403.
- Jiříčková A, Jankovský O, Sofer Z, Sedmidubský D. Synthesis and applications of graphene oxide. Materials 2022; 15(3): 920. doi: 10.3390/ma15030920.
- Yu W, Sisi L, Haiyan Y, Jie L. Progress in the functional modification of graphene/graphene oxide: A review. RSC Advances 2020; 10(26): 15328–15345. doi: 10.1039/d0ra01068e.
- Shahriary L, Athawale AA. Graphene oxide synthesized by using modified Hummers approach. International Journal of Renewable Energy and Environmental Engineering 2014; 2(1): 58–63.
- Moosa AA, Abed MS. Graphene preparation and graphite exfoliation. Turkish Journal of Chemistry 2021; 45(3): 493–519. doi: 10.3906/kim-2101-19.
- Chua CK, Pumera M. Chemical reduction of graphene oxide: A synthetic chemistry viewpoint. Chemical Society Reviews 2014; 43(1): 291–312. doi: 10.1039/c3cs60303b.
- Brodie BC. On the atomic weight of graphite. Philosophical Transactions of the Royal Society of London 1859; 149(149): 249–259. doi: 10.1098/rstl.1859.0013.
- Ojha K, Anjaneyulu O, Ganguli AK. Graphene-based hybrid materials: Synthetic approaches and properties. Current Science 2014; 107: 397–418.
- Hummers WS, Offeman RE. Preparation of graphitic oxide. Journal of the American Chemical Society 1958; 80: 1339. doi: 10.1021/ja01539a017.
- Lee J, Kim J, Kim S, Min DH. Biosensors based on graphene oxide and its biomedical application. Advanced Drug Delivery Reviews 2016; 105: 275–287. doi: 10.1016/j.addr.2016.06.001.
- Marcano DC, Kosynkin DV, Berlin JM, et al. Improved synthesis of graphene oxide. ACS Nano 2010; 4(8): 4806–4814. doi: 10.1021/nn1006368.
- Ma J, Liu R, Wang X, et al. Crucial role of lateral size for graphene oxide in activating macrophages and stimulating pro-inflammatory responses in cells and animals. ACS Nano 2015; 9(10): 10498–10515. doi: 10.1021/acsnano.5b04751.
- Smith AT, LaChance AM, Zeng S, et al. Synthesis, properties, and applications of graphene oxide/reduced graphene oxide and their nanocomposites. Nano Materials Science 2019; 1(1): 31–47. doi: 10.1016/j.nanoms.2019.02.004.
- Zhao J, Pei S, Ren W, et al. Efficient preparation of large-area graphene oxide sheets for transparent conductive films. ACS Nano 2010; 4(9): 5245–5252. doi: 10.1021/nn1015506.
- Cao W, He L, Cao W, et al. Recent progress of graphene oxide as a potential vaccine carrier and adjuvant. Acta Biomaterialia 2020; 112: 14–28. doi: 10.1016/j.actbio.2020.06.009.
- Yunus MA, Ramli MM, Osman NH, Mohamed R. Stimulation of innate and adaptive immune cells with graphene oxide and reduced graphene oxide affect cancer progression. Archivum Immunologiae et Therapiae Experimentalis 2021; 69(1): 20. doi: 10.1007/s00005-021-00625-6.
- Zhi X, Fang H, Bao C, et al. The immunotoxicity of graphene oxides and the effect of PVP-coating. Biomaterials 2013; 34(21): 5254–5261. doi: 10.1016/j.biomaterials.2013.03.024.
- Wang W, Li Z, Duan J, et al. In vitro enhancement of dendritic cell-mediated anti-glioma immune response by graphene oxide. Nanoscale Research Letters 2014; 9(1): 311. doi: 10.1186/1556-276X-9-311.
- Li H, Fierens K, Zhang Z, et al. Spontaneous protein adsorption on graphene oxide nanosheets allowing efficient intracellular vaccine protein delivery. ACS Applied Materials & Interfaces 2016; 8(2): 1147–1155. doi: 10.1021/acsami.5b08963.
- Yang Z, Pan Y, Chen T, et al. Cytotoxicity and immune dysfunction of dendritic cells caused by graphene oxide. Frontiers in Pharmacology 2020; 11: 1206. doi: 10.3389/fphar.2020.01206.
- Parker H, Gravagnuolo AM, Vranic S, et al. Graphene oxide modulates dendritic cell ability to promote T cell activation and cytokine production. Nanoscale 2022; 14(46): 17297–17314. doi: 10.1039/d2nr02169b.
- Zhou Q, Gu H, Sun S, et al. Large-sized graphene oxide nanosheets increase DC-T-cell synaptic contact and the efficacy of DC vaccines against SARS-CoV-2. Advanced Materials 2021; 33(40): e2102528. doi: 10.1002/adma.202102528.
- Zheng T, Gao Y, Deng X, et al. Comparisons between graphene oxide and graphdiyne oxide in physicochemistry biology and cytotoxicity. ACS Applied Materials & Interfaces 2018; 10(39): 32946–32954. doi: 10.1021/acsami.8b06804.
- Jia PP, Sun T, Junaid M, et al. Nanotoxicity of different sizes of graphene (G) and graphene oxide (GO) in vitro and in vivo. Environmental Pollution 2019; 247: 595–606. doi: 10.1016/j.envpol.2019.01.072.
- Gu Y, Guo Y, Wang C, et al. A polyamidoamne dendrimer functionalized graphene oxide for DOX and MMP-9 shRNA plasmid co-delivery. Materials Science and Engineering: C 2017; 70: 572–585. doi: 10.1016/j.msec.2016.09.035.
- Wang B, Su X, Liang J, et al. Synthesis of polymer-functionalized nanoscale graphene oxide with different surface charge and its cellular uptake, biosafety and immune responses in Raw264.7 macrophages. Materials Science and Engineering: C 2018; 90: 514–522. doi: 10.1016/j.msec.2018.04.096.
- Xu L, Xiang J, Liu Y, et al. Functionalized graphene oxide serves as a novel vaccine nano-adjuvant for robust stimulation of cellular immunity. Nanoscale 2016; 8(6): 3785–3795. doi: 10.1039/c5nr09208f.
- Wang X, Cao F, Yan M, et al. Alum-functionalized graphene oxide nanocomplexes for effective anticancer vaccination. Acta Biomaterialia 2019; 83: 390–399. doi: 10.1016/j.actbio.2018.11.023.
- Liao Y, Zhou X, Fu Y, Xing D. Graphene oxide as a bifunctional material toward superior RNA protection and extraction. ACS Applied Materials & Interfaces 2018; 10(36): 30227–30234. doi: 10.1021/acsami.8b12522.
- Rhazouani A, Gamrani H, El Achaby M, et al. Synthesis and toxicity of graphene oxide nanoparticles: A literature review of in vitro and in vivo studies. BioMed Research International 2021; 2021: 5518999. doi: 10.1155/2021/5518999.
- Liang J, Zhao X. Nanomaterial-based delivery vehicles for therapeutic cancer vaccine development. Cancer Biology & Medicine 2021; 18(2): 352–371. doi: 10.20892/j.issn.2095-3941.2021.0004.
- Reina G, González-Domínguez JM, Criado A, et al. Promises, facts and challenges for graphene in biomedical applications. Chemical Society Reviews 2017; 46(15): 4400–4416. doi: 10.1039/c7cs00363c.