Article
Determination of Optimal Designs for Geothermal Energy Piles in the Soil Supporting a Multi-storey Building
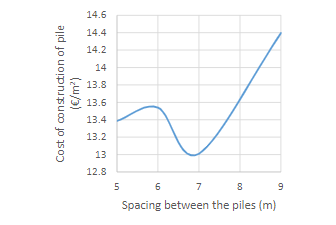
Downloads
Download

This work is licensed under a Creative Commons Attribution 4.0 International License.
Copyright
The authors shall retain the copyright of their work but allow the Publisher to publish, copy, distribute, and convey the work.
License
New Energy Exploitation and Application (NEEA) publishes accepted manuscripts under Creative Commons Attribution 4.0 International (CC BY 4.0). Authors who submit their papers for publication by New Energy Exploitation and Application (NEEA) agree to have the CC BY 4.0 license applied to their work, and that anyone is allowed to reuse the article or part of it free of charge for any purpose, including commercial use. As long as the author and original source is properly cited, anyone may copy, redistribute, reuse and transform the content.
The article presents the optimal design of geothermal energy piles supporting a multi-story building at different pile spacing. The optimization model OPTPILE, based on the construction cost of the single pile, was used for this purpose. It was provided with geotechnical and structural design constraints that satisfy the requirements of building codes. The optimal design of a geothermal energy pile was studied for a 10-storey building with different pile spacing. So far, only a single energy pile has been optimized and therefore the spacing between the piles has not yet been considered in the design. However, in this work, pile spacing is taken into account by considering the entire load distribution of the multi-story building. A 3D beam-slab frame was created to determine the pile loads. The recommendation for the optimal design of geothermal energy pile spacing for a 10-storey building was developed. The results show that the optimal pile spacing (square distribution) for a 10-storey building is about 7 m. The construction cost for all thermal pile foundations and concrete structural components for a 10-storey building is estimated to be 101.1 €/m2 . The optimal architecturally reasonable spacing of piles with a square distribution for a 10-storey building is 8.5 m. In this case, the cost of the concrete structural elements of the building and the piles increases by 1% to 102.1 €/m2 . The cost of installing the heating pipes in the pile is about 1 €/m2 .
Keywords:
Geothermal energy piles Structural design Optimization Multi-storey buildingReferences
- Han, C., Yu, X., 2018. An innovative energy pile technology to expand the viability of geothermal bridge deck snow melting for different United States regions: Computational assisted feasibility analyses. Renewable Energy. 123, 417-427.
- Ghasemi-Fare, O., Basu, P., 2016. Predictive assessment of heat exchange performance of geothermal piles. Renewable Energy. 86, 1178-1196.
- Han, C., Yu, X., 2017. Feasibility of geothermal heat exchanger pile-based bridge deck snow melting system: A simulation based analysis. Renewable Energy. 101, 214-224.
- Jalaluddin, A., Miyara, K., Tsubaki, S., et al., 2011. Experimental study of several types of ground heat exchanger using a steel pile foundation. Renewable Energy. 36, 764-771.
- Bayer, P., Attard, G., Blum, P., et al., 2019. The geothermal potential of cities. Renewable and Sustainable Energy Reviews. 106, 17-30. DOI: https://doi.org/10.1016/j.rser.2019.02.019
- Maragna, C., Loveridge, F., 2019. A resistive-capacitive model of pile heat exchangers with an application to thermal response tests interpretation. Renewable Energy. 138, 891-910. DOI: https://doi.org/10.1016/j.renene.2019.02.012
- Yazdani, S., Helwany, S., Olgun, G., 2019. Influence of temperature on soil-pile interface shear strength. Geomechanics for Energy & the Environment. 18, 69-78.
- EN 1997-1:2007, 2007. Eurocode 7: Geotechnical design - Part 1: General rules.
- Poulos, H.G., 1989. Pile behaviour: theory and application. Géotechnique. 39, 363-415.
- EN 1992-1:2004, 2004. Eurocode 2: Design of concrete structures- Part 1: General rules and rules for buildings.
- Ray, S.S., 1995. Reinforced Concrete Analysis & Design. 1 edition, Wiley-Blackwell.
- Rotta Loria, A.F., Bocco, M., Garbellini, C., et al., 2019. The role of thermal loads in the performance-based design of energy piles. Geomechanics for Energy and the Environment. pp. 100153. DOI: https://doi.org/10.1016/j.gete.2019.100153
- Fromentin, G., Pahud, A., Sarlos D., 1998. Heating and cooling systems with heat exchanger piles. Conference proceedings or Presentation at a conference. pp. 230-234.
- Ghasemi-Fare, O., Basu, P., 2013. A practical heat transfer model for geothermal piles. Energy and Buildings. 66, 470-479. DOI: https://doi.org/10.1016/j.enbuild.2013.07.048
- Stewart, M.A., McCartney, J.S., 2014. Centrifuge Modeling of Soil-Structure Interaction in Energy Foundations. Journal of Geotechnical and Geoenvironmental. 140.
- Saggu, R., Chakraborty, T., 2017. Thermomechanical Analysis and Parametric Study of Geothermal Energy Piles in Sand. International Journal of Geomechanics. ASCE, 1-17.
- Knellwolf, C., Peron, H., Laloui, L., 2011. Geotechnical Analysis of Heat Exchanger Piles. Journal Of Geotechnical And Geoenvironmental Engineering. 137, 890-902.
- Laloui, L., Nuth, M., Vulliet, L., 2006. Experimental and numerical investigations of the behaviour of a heat exchanger pile. International Journal for Numerical and Analytical Methods. 30, 763-781.
- GSHP Association, 2012. Thermal pile design, installation and materials standards, Gr. Source Heat Pump Assoc. Milt. Keynes. 82.
- Viswanathan, J., Grossmann, I.E., 1990. A combined penalty function and outer-approximation method for MINLP optimization. Computers & Chemical Engineering. 14, 769-782.
- Jelušič, P., Žlender, B., 2018. Determining optimal designs for conventional and geothermal energy piles. Renewable Energy.
- EN 1998-1:2005, 2005. Eurocode 8: Design of structures for earthquake resistance - Part 1: General rules, seismic actions and rules for buildings.
- SAP2000 Version 14, 2010. Basic Analysis Reference, Computers & Structures, Inc., Berkeley, California, USA.
- Jelušič, P., Žlender, B., Dolinar, B., 2016. NLP optimization model as a failure mechanism for geosynthetic reinforced slopes subjected to pore-water pressure. International Journal of Geomechanics. 16.
- Jelušič, P., 2015. Soil compaction optimization with soft constrain. Journal of Intelligent and Fuzzy Systems. 29.
- Jelušič, P., Žlender, B., 2017. Optimal design of piled embankments with basal reinforcement. Geosynthetics International. pp. 1-30.