Article
Techno-Economic Analysis of Heat-Assisted Hydrogen Production from Nuclear Power
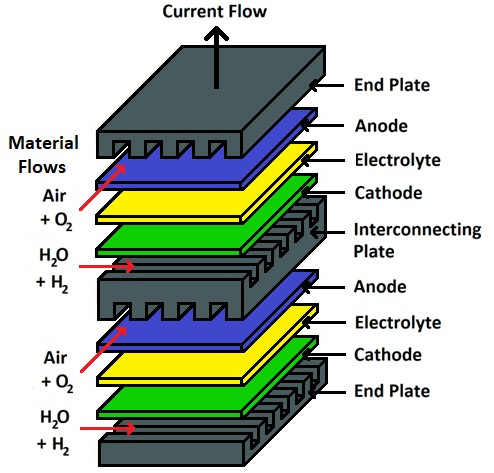
Downloads
Download


This work is licensed under a Creative Commons Attribution 4.0 International License.
Copyright
The authors shall retain the copyright of their work but allow the Publisher to publish, copy, distribute, and convey the work.
License
New Energy Exploitation and Application (NEEA) publishes accepted manuscripts under Creative Commons Attribution 4.0 International (CC BY 4.0). Authors who submit their papers for publication by New Energy Exploitation and Application (NEEA) agree to have the CC BY 4.0 license applied to their work, and that anyone is allowed to reuse the article or part of it free of charge for any purpose, including commercial use. As long as the author and original source is properly cited, anyone may copy, redistribute, reuse and transform the content.
To play a full role in decarbonisation, hydrogen must be produced economically at scale; the role of nuclear power is interesting to national governments as it is capable of supplying both low-carbon electricity and high-quality heat. Depending on the hydrogen production technology choice, a plant may require electricity and/or heat input. This techno-economic evaluation considers not only the costs of the hydrogen plant itself but also the costs of the power supply it requires. This paper calculates cost estimates for HTSE (High Temperature Steam Electrolysis) coupled with nuclear heat and electricity, and a thermochemical SI (Sulphur Iodine) cycle coupled with nuclear heat, based on the predictions of technical process models. These estimates are then compared to estimates made elsewhere for the costs of using wind with low temperature liquid water electrolysis, and steam methane reformation with carbon capture. This analysis led to the identification of the conditions under which nuclear-heat-coupled hydrogen production would be competitively priced. Estimates for nuclear-coupled LCOH2 (levelised cost of hydrogen) in 2050 range from 2.14 to 1.24 £/kg for HTSE, and from 2.88 to 0.89 £/kg for the SI cycle. There is still a great deal of uncertainty around the efficiency of SI and how it may improve over time, and this limits the accuracy to which the LCOH2 can be predicted.
Keywords:
nuclear hydrogen production economics technology readiness levels steam electrolysis thermochemicalReferences
- Staffell, I.; Scamman, D.; Velazquez, A.; Balcombe, P.; Dodds, P.E.; Ekins, P.; Shah, N.; Ward, K.R. The Role of Hydrogen and Fuel Cells in the Global Energy System. Energy Environ. Sci. 2019, 12, 463–491.
- Fast-Tracking Nuclear Hydrogen: IAEA to Develop Roadmap for Commercial Deployment. Available online: https://www.iaea.org/newscenter/news/fast-tracking-nuclear-hydrogen-iaea-to-develop-roadmap-for-commercial-deployment (accessed on 26 March 2024).
- Constantin, A. Nuclear Hydrogen Projects to Support Clean Energy Transition: Updates on International Initiatives and IAEA Activities. Int. J. Hydrog. Energy 2024, 54, 768–779.
- Hydrogen Production with Operating Nuclear Power Plants. Available online: https://www.iaea.org/sites/default/files/2023_h2_booklet_web.pdf (accessed on 26 April 2024).
- Bay Hydrogen Hub—Hydrogen-4-Hanson: Department for Energy Security and Net Zero (DESNEZ) Industrial Hydrogen Accelerator Stream 2A Feasibility Study. Available online: https://assets.publishing.service.gov.uk/government/uploads/system/uploads/attachment_data/file/1161490/iha-22-edf-final-feasibility-report.pdf (accessed on 26 February 2024).
- Hydrogen Projects Database. Available online: https://www.iea.org/data-and-statistics/data-product/hydrogen-production-and-infrastructure-projects-database (accessed on 26 April 2024).
- Levelised Cost of Hydrogen Maps. Available online: https://www.iea.org/data-and-statistics/data-tools/levelised-cost-of-hydrogen-maps (accessed on 26 April 2024).
- International Atomic Energy Agency. Examining the Technoeconomics of Nuclear Hydrogen Production and Benchmark Analysis of the IAEA HEEP software. IAEA TECDOC 1859, 2018.
- Naterer, G.F.; Dincer, I.; Zamfirescu, C. Hydrogen Production from Nuclear Energy; Springer International Publishing AG: Midtown Manhattan, New York City, USA, 2013.
- UK Hydrogen Strategy. Available online: https://assets.publishing.service.gov.uk/media/64c7e8bad8b1a70011b05e38/UK-Hydrogen-Strategy_web.pdf (accessed on 26 April 2024).
- Shahabuddin, M.; Alim, M.A.; Alam, T.; Mofijur M.; Ahmed, S.F.; Perkins G.A. Critical Review on the Development and Challenges of Concentrated Solar Power Technologies. Sustainable Energy Technol. Assess. 2021, 47, 101434.
- El-Emam R.S.; Özcan, H. Comprehensive Review on the Technoeconomics of Sustainable Large-Scale Clean Hydrogen Production. J. Cleaner Prod. 2019, 220, 593–609.
- Beyond Electricity: The Economics of Nuclear Cogeneration. Available online: https://www.oecd-nea.org/jcms/pl_71699/beyond-electricity-the-economics-of-nuclear-cogeneration (accessed on 25 March 2024).
- Gabriel, K.S.; El-Emam, R.S.; Zamfirescu, C. Technoeconomics of large-scale clean hydrogen production—A review. Int. J. Hydrogen Energy 2022, 47, 30788–30798.
- H2A: Hydrogen Analysis Production Methods. Available online: https://www.nrel.gov/hydrogen/h2a-production-models.html (accessed on 26th April 2024).
- GEN IV International Forum. Available online: https://www.gen-4.org/gif/jcms/c_9509/tools (accessed on 26 April 2024).
- Frick, K.; Wendt, D.; Talbot, P.; Rabiti, C.; Boardman, R. Technoeconomic Assessment of Hydrogen Cogeneration via High Temperature Steam Electrolysis with a Light-Water Reactor. Appl. Energy 2022, 306, 118044.
- Bhattacharyya, R.; Singh, K.K.; Bhanja, K.; Grover, R.B. Assessing Techno-Economic Uncertainties in Nuclear Power-to-X Processes: The Case of Nuclear Hydrogen Production via Water Electrolysis. Int. J. hydrog. Energy 2023, 48, 14149–14169.
- Epiney, A.; Rabiti, C.; Talbot, P.; Alfonsi, A. Economic Analysis of a Nuclear Hybrid Energy System in a Stochastic Environment including Wind Turbines in an Electricity Grid. Appl. Energy 2020, 260, 114227.
- Antony, A.; Maheshwari, N.K.; Rama Rao, A. A Generic Methodology to Evaluate Economics of Hydrogen Production Using Energy from Nuclear Power Plants. Int. J. Hydrog. Energy 2017, 42, 25813–25823.
- Slavin, B.; Wang, R.; Roy, D.; Ling-Chin, J.; Roskilly, A.P. Techno-Economic Analysis of Direct Air Carbon Capture and Hydrogen Production Integrated with a Small Modular Reactor. Appl. Energy 2024, 356, 122407.
- IEAGHG Technical Report, “Techno-Economic Evaluation of SMR Based Standalone (Merchant) Hydrogen Plant with CCS”, February 2017. Available online: https://ieaghg.org/exco_docs/2017-02.pdf (accessed on 29 April 2024).
- El-Emam, R.S.; Khamis, I. International Collaboration in the IAEA Nuclear Hydrogen Production Program for Benchmarking of HEEP. Int. J. Hydrog. Energy 2016, 42, 3566–3571.
- Khamis, I.; Malshe, U.D. HEEP: A new tool for the economic evaluation of hydrogen economy. Int. J. Hydrog. Energy 2010, 35, 8398–8406.
- El-Emam, R.S.; Ozcan, H.; Dincer, I. Comparative Cost Evaluation of Nuclear Hydrogen Production Methods with the Hydrogen Economy Evaluation Program (HEEP). Int. J. Hydrog. Energy 2015, 40, 11168–11177.
- Lovering, J.R.; Yip, A.; Nordhaus, T. Historical construction costs of global nuclear power reactors. Energy Policy 2016, 91, 371–382.
- Hydrogen Production Costs. Available online: https://www.gov.uk/government/publications/hydrogen-production-costs-2021 (accessed on 26 April 2024).
- Civil Nuclear: Roadmap to 2050. Available online: https://assets.publishing.service.gov.uk/media/65c0e7cac43191000d1a457d/6.8610_DESNZ_Civil_Nuclear_Roadmap_report_Final_Web.pdf (accessed on 26 April 2024).
- Perfiliev, M.V. Problems of High Temperature Electrolysis of Water Vapour. Int. J. Hydrog. Energy 1994, 19. 227–230.
- Ni, M.; Leung, M.K.H.; Leung, D.Y.C. An Electrochemical Model of a Solid Oxide Steam Electrolyser for Hydrogen Production. Chem. Eng. Tech. 2006, 29, 636–642.
- Ferguson, J.R.; Fiard, J.M.; Herbin, R. Three-dimensional numerical simulation for various geometries of solid oxide fuel cells. Power Sources 1996, 58, 109–122.
- Solid Oxide Electrolysis System Demonstration. Available online: https://www.hydrogen.energy.gov/docs/hydrogenprogramlibraries/pdfs/review23/ta039_ghezel-ayagh_2023_o-pdf.pdf (accessed on 26 April 2024).
- Offshore Wind and Hydrogen: Solving the Integration Challenge. Available online: https://ore.catapult.org.uk/wp-content/uploads/2020/09/Solving-the-Integration-Challenge-ORE-Catapultr.pdf (accessed on 26 April 2024).
- HyNet Low Carbon Hydrogen Plant. Available online: https://assets.publishing.service.gov.uk/media/5e4ac453ed915d4fff2dbf04/HS384_-_Progressive_Energy_-_HyNet_hydrogen.pdf (accessed on 26 April 2024).
- Wang, L.J.; Zhang, P.; Chen, S.Z.; Xu, J.M. Overview of the Development of Catalysts for HI Decomposition in the Iodine-Sulfur Thermochemical Cycle at INET. Nucl. Eng. Des. 2014, 271, 60–63.
- Zhang, K.; Bao, W.; Chang, L.; Wang, H. A Review of Recent Researches on Bunsen Reaction for Hydrogen Production via S–I Water and H2S Splitting Cycles. J. Energy Chem. 2019, 33, 46–58.
- Preliminary Economic Assessment of GW Scale Nuclear Enabled Hydrogen Production. Available online: https://www.nnl.co.uk/wp-content/uploads/2022/03/Preliminary-Economic-Assessment-of-Nuclear-Enabled-Hydrogen-Production-Final-Approved.pdf (accessed on 26 April 2024).
- Brown, N.R.; Oh, S; Revankar, S.T; Vierow, K; Rodriguez, S.; Cole, R.; Gauntt, R. Simulation of Sulfur-Iodine Thermochemical Hydrogen Production Plant Coupled to High-Temperature Heat Source. Nucl. Technol. 2009, 167, 95–106.