Article
Thermal Conductivity of CaSrFe0.75Co0.75Mn0.5O6-δ
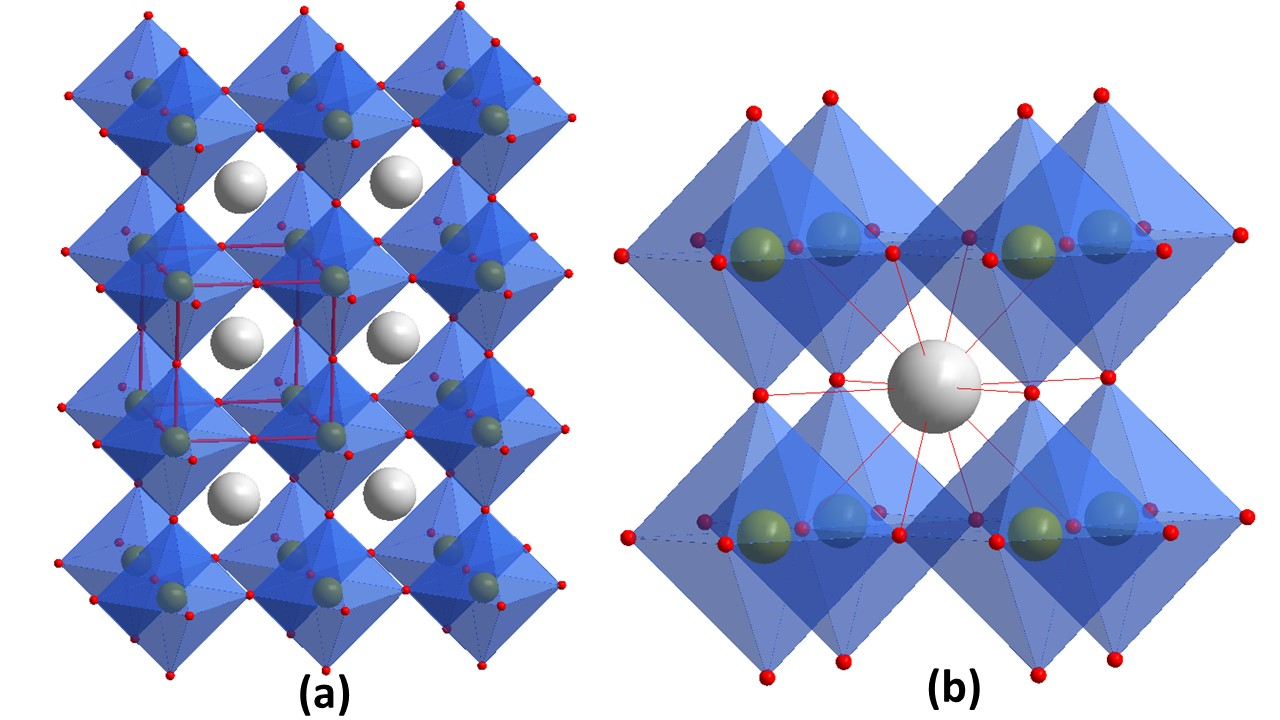
Downloads
Download

This work is licensed under a Creative Commons Attribution 4.0 International License.
Copyright
The authors shall retain the copyright of their work but allow the Publisher to publish, copy, distribute, and convey the work.
License
New Energy Exploitation and Application (NEEA) publishes accepted manuscripts under Creative Commons Attribution 4.0 International (CC BY 4.0). Authors who submit their papers for publication by New Energy Exploitation and Application (NEEA) agree to have the CC BY 4.0 license applied to their work, and that anyone is allowed to reuse the article or part of it free of charge for any purpose, including commercial use. As long as the author and original source is properly cited, anyone may copy, redistribute, reuse and transform the content.
CaSrFe0.75Co0.75Mn0.5O6-δ, an oxygen-deficient perovskite, had been reported for its better electrocatalytic properties of oxygen evolution reaction. It is essential to investigate different properties such as the thermal conductivity of such efficient functional materials. The thermal conductivity of CaSrFe0.75Co0.75Mn0.5O6-δ is a critical parameter for understanding its thermal transport properties and potential applications in energy conversion and electronic devices. In this study, the authors present an investigation of the thermal conductivity of CaSrFe0.75Co0.75Mn0.5O6-δ at room temperature for its thermal insulation property study. Experimental measurement was conducted using a state-of-the-art thermal characterization technique, Thermtest thermal conductivity meter. The thermal conductivity of CaSrFe0.75Co0.75Mn0.5O6-δ was found to be 0.724 W/m/K at 25 °C, exhibiting a notable thermal insulation property i.e., low thermal conductivity.
Keywords:
XRD solid-state reaction thermal conductivity perovskite oxidesReferences
- Cohen, R.E. Origin of ferroelectricity in perovskite oxides. Nature 1992, 358, 136–138.
- Tyunina, M. Oxygen vacancies in perovskite oxide piezoelectrics. Materials 2020, 13, 5596.
- Kim, M.; McNally, G.M.; Kim, H.H.; Oudah, M.; Gibbs, A.S.; Manuel, P.; Green, R.J.; Sutarto, R.; Takayama, T.; Yaresko, A. et al. Superconductivity in (Ba, K) SbO3. Nat. Mater. 2022, 21, 627–633.
- Hona, R.K.; Ramezanipour, F. Remarkable oxygen‐evolution activity of a perovskite oxide from the Ca2−xSrxFe2O6−δ series. Angew. Chem. Int. Ed. 2019, 58, 2060–2063.
- Hona, R.K.; Karki, S.B.; Ramezanipour, F. Oxide electrocatalysts based on earth-abundant metals for both hydrogen-and oxygen-evolution reactions. ACS Sustainable Chem. Eng. 2020, 8(31), 11549–11557.
- Xu, X.; Wang, W.; Zhou, W.; Shao, Z. Recent advances in novel nanostructuring methods of perovskite electrocatalysts for energy‐related applications. Small Methods 2018, 2, 1800071.
- Shu, L.; Sunarso, J.; Hashim, S.S.; Mao, J.; Zhou, W.; Liang, F. Advanced perovskite anodes for solid oxide fuel cells: A review. Int. J. Hydrog. Energy. 2019, 44, 31275–31304.
- Takeguchi, T.; Yamanaka, T.; Takahashi, H.; Watanabe, H.; Kuroki, T.; Nakanishi, H.; Orikasa, Y.; Uchimoto, Y.; Takano, H.; Ohguri, N.; et al. Layered perovskite oxide: A reversible air electrode for oxygen evolution/reduction in rechargeable metal-air batteries. J. Am. Chem. Soc. 2013, 135, 11125–11130.
- Hona, R.K.; Thapa, A.K.; Ramezanipour, F. An anode material for lithium‐ion batteries based on oxygen‐deficient perovskite Sr2Fe2O6−δ. ChemistrySelect. 2020, 5, 5706–5711.
- Hona, R.K.; Karki, S.B.; Cao, T.; Mishra, R.; Sterbinsky, G.E.; Ramezanipour, F. Sustainable oxide electrocatalyst for hydrogen-and oxygen-evolution reactions. ACS Catal. 2021, 11, 14605–14614.
- Hona, R.K.; Karki, S.B.; Dhaliwal, G.S.; Guinn, M.; Ramezanipour, F. High thermal insulation properties of A2FeCoO6−δ (A = Ca, Sr). J. Mater. Chem. C. 2022, 10, 12569–12573.
- Karki, S.B.; Hona, R.K.; Ramezanipour, F. Effect of structure on sensor properties of oxygen-deficient perovskites, A2BB′O5 (A = Ca, Sr; B = Fe; B′ = Fe, Mn) for oxygen, carbon dioxide and carbon monoxide sensing. J. Electron. Mater. 2020, 49, 1557–1567.
- He, J.; Xu, X.; Li, M.; Zhou, S.; Zhou, W. Recent advances in perovskite oxides for non-enzymatic electrochemical sensors: A review. Anal. Chim. Acta. 2023, 1251, 341007.
- Sahare, S.; Kumar, M.; Ghoderao, P.; Aepuru, R.; Lee, S.L.; Yun, J.H. Oxide perovskites and their derivatives for photovoltaics applications. In Advanced Ceramics for Energy and Environmental Applications, 1st ed.; CRC Press: Boca Raton, US, 2021.
- Xu, X.; Pan, Y.; Zhong, Y.; Ran, R.; Shao, Z. Ruddlesden–Popper perovskites in electrocatalysis. Mater. Horiz. 2020, 7, 2519–2565.
- Martinson, A.; Guinn, M.; Hona, R.K. The crystal structure study of CaSrFe0.75Co0.75Mn0.5O6−δ by neutron diffraction. J. Mater. Sci. Chem. Eng. 2024, 12, 29–35.
- Zhang, M.; Zou, P.; Jeerh, G.; Sun, B.; Walker, M.; Tao, S. Oxygen vacancy‐rich La0.5Sr1.5Ni0.9Cu0.1O4–δ as a high‐performance bifunctional catalyst for symmetric ammonia electrolyzer. Adv. Funct. Mater. 2022, 32, 2204881.
- Wang, S.; Han, P.; Zhao, Y.; Sun, W.; Wang, R.; Jiang, X.; Wu, C.; Sun, C.; Wei, H. Oxygen-vacancy-mediated LaFe1−xMnxO3−δ perovskite nanocatalysts for degradation of organic pollutants through enhanced surface ozone adsorption and metal doping effects. Nanoscale. 2021, 13, 12874–12884.
- Maire, J.; Anufriev, R.; Yanagisawa, R.; Ramiere, A.; Volz, S.; Nomura, M. Heat conduction tuning by wave nature of phonons. Sci. Adv. 2017, 3, e1700027.
- Snyder, G.J.; Toberer, E.S. Complex thermoelectric materials. Nature Mater. 2008, 7, 105–114.
- Delorme, F.; Chen, C.; Schoenstein, F.; Jaber, N.; Jean, F.; Bah, M.; Simon, Q.; Chartier, T.; Laffez, P.; Monot-Laffez, I.; et al. Low intrinsic thermal conductivity of Spark Plasma Sintered dense KNbO3 and NaNbO3 perovskite ceramics. Thermochim. Acta. 2021, 695, 178807.
- Bocher, L.; Aguirre, M.H.; Logvinovich, D.; Shkabko, A.; Robert, R.; Trottmann, M.; Weidenkaff, A. CaMn1−xNbxO3 (x ≤ 0.08) perovskite-type phases as promising new high-temperature n-type thermoelectric materials. Inorg. Chem. 2008, 47(18), 8077–8085.
- Zhang, P.; Gong, L.; Lou, Z.; Xu, J.; Cao, S.; Zhu, J.; Yan, H.; Gao, F. Reduced lattice thermal conductivity of perovskite-type high-entropy (Ca0.25Sr0.25Ba0.25RE0.25) TiO3 ceramics by phonon engineering for thermoelectric applications. J. Alloys Compd. 2022, 898, 162858.
- Wu, T.; Gao, P. Development of perovskite-type materials for thermoelectric application. Materials 2018, 11, 999.
- Vijay, V.; Harish, S.; Archana, J.; Navaneethan, M. Synergistic effect of grain boundaries and phonon engineering in Sb substituted Bi2Se3 nanostructures for thermoelectric applications. J. Colloid Interface Sci. 2022, 612, 97–110.
- Lou, Z.; Zhang, P.; Zhu, J.; Gong, L.; Xu, J.; Chen, Q.; Reece, M.J.; Yan, H.X.; Gao, F. A novel high-entropy perovskite ceramics Sr0.9La0.1(Zr0.25Sn0.25Ti0.25Hf0.25) O3 with low thermal conductivity and high Seebeck coefficient. J. Eur. Ceram. Soc. 2022, 42, 3480–3488.
- Zheng, W.; Cao, W.; Wang, Z.; Deng, H.; Shi, J.; Xiong, R. Improvement of the thermoelectric properties of a MoO3 monolayer through oxygen vacancies. Beilstein J. Nanotechnol. 2019, 10, 2031–2038.
- Wang, Y.; Sui, Y.; Wang, X.; Su, W.; Liu, X.; Fan, H.J. Thermal conductivity of electron-doped CaMnO3 perovskites: Local lattice distortions and optical phonon thermal excitation. Acta Mater. 2010, 58, 6306–6316.
- Rahman, J.U.; Nam, W.H.; Van Du, N.; Rahman, G.; Rahman, A.U.; Shin, W.H.; Seo, W.S.; Kim, M.H.; Lee, S. Oxygen vacancy revived phonon-glass electron-crystal in SrTiO3. J. Eur. Ceram. Soc. 2019, 39, 358–365.
- Schlichting, K.W.; Padture, N.P.; Klemens, P.G. Thermal conductivity of dense and porous yttria-stabilized zirconia. J. Mater. Sci. 2001, 36, 3003–3010.